Air quality from Greeley to Ghana
You may have noticed that the air quality in parts of Colorado has been worse than usual for the past few days. Wildfires burning across the U.S. (although notably not in Colorado) have caused smokey skies of late. This far-flung smoke highlights how pollution in one area isn’t limited to or explained by simple factors such as the number of cars there or a particularly gross factory next door. Bridget Menasche writes about how CU Boulder scientists, in collaboration with scientists all over the world, are studying air quality with new technology and local help.
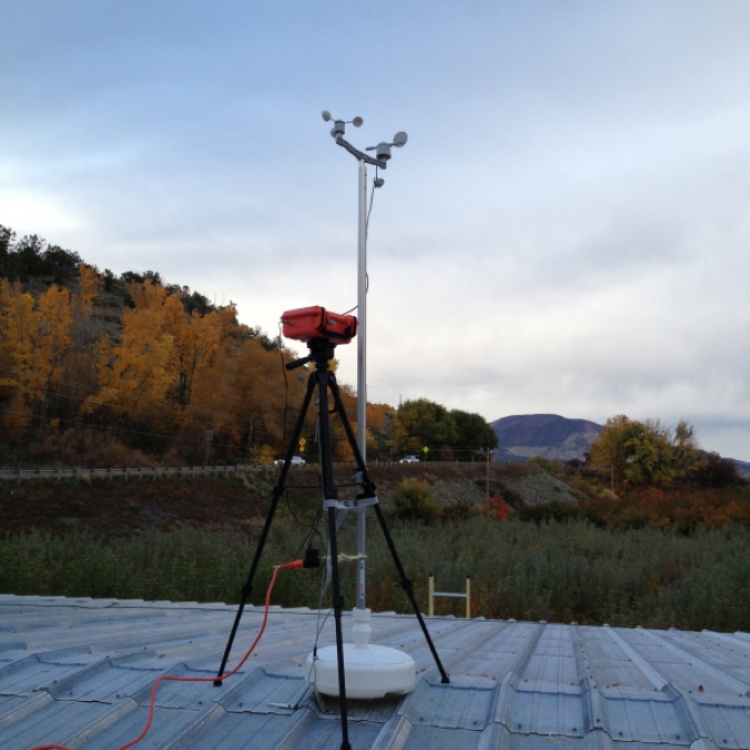
A Y-Pod set up by Ashley Collier-Oxandale in the Hannigan lab. Photo credit: Ashley Collier-Oxandale
Air pollution persistently influences climate change and public health. In Boulder, we see it most when wildfire smoke crawls along the Front Range and turns the sun into a rising blood orange. Visible smoke or haze rarely tells the whole story—unlike diesel spilled in Boulder Creek, changes in air quality are often impossible to see. Spikes in greenhouse gas emissions from oil wells and hydraulic fracturing sites wisp through the air, invisible. Over time, however, changes in air quality become visible through respiratory disease and climate change.
We walk through the atmosphere blind to these changes. But what if you could set up monitoring equipment on your roof or near a source of greenhouse gasses in your neighborhood? The development of the Y-Pod from the Hannigan lab at CU Boulder makes this local monitoring possible across Colorado and around the world.
For the past few years, high school students in Greeley, Weld County, and Delta County Colorado have spent their spring semester measuring air quality with Y-Pods. As part of an initiative called the Air Quality InQuiry program (AQ-IQ), the students use these (big) tackle-box-sized instruments to sense methane, carbon dioxide, ground-level ozone, and volatile organic compounds like car exhaust, benzenes, paint solvents, and perfume.
Monitoring for the Masses
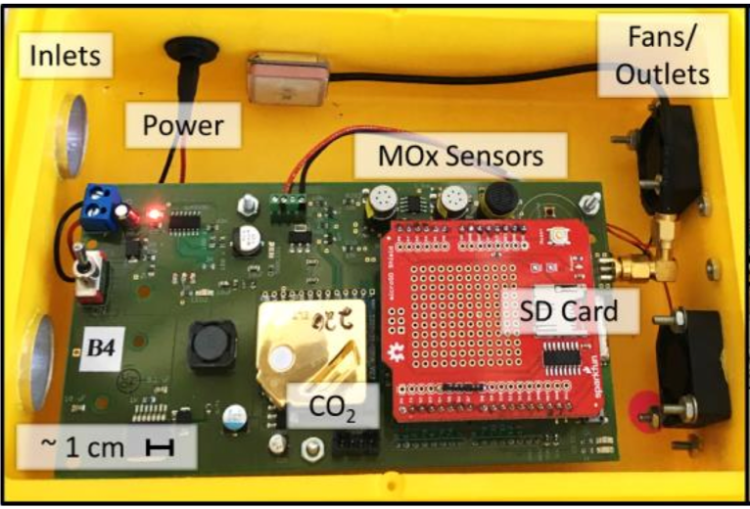
Labeled photo of a Y-Pod interior. Figure 1 from “Assessing a low-cost methane sensor quantification system for use in complex rural and urban environments.”
The Y-Pod’s thick plastic case houses a printed circuit board—think the motherboard in a gamer buddy’s desktop—containing metal oxide (MOx) sensors attached to a data logger. Each sensor contains an electrolyte that interacts specifically with the gas being measured to generate an electrical current. That current can later be analyzed to get time-sensitive estimates of greenhouse gas and pollutant concentrations in the air. Plug the Y-Pod into a car battery or wall outlet, let it warm up for half an hour, and the sensors will log multiple observations per minute, which are then stored on a mini-SD card for later analysis.
Traditional sensors use optical methods to measure gas concentrations; they are more accurate but also much more expensive. The Y-Pod was designed to be cheap. A case holding two pods and a power supply cost about a thousand dollars to produce, a tenth of the cost of other sensors. Although the instrument can’t be ordered at that low, low price in easy payments of $19.95, it does make it possible for local communities and other small organizations to afford to do their own air-quality research.
The original Pod model was designed to research emissions across oil and gas development sites as part of the NSF AirWaterGas sustainability research network. In 2013, high school teachers in Colorado’s Delta County got involved, set up projects with their students, and started a collaboration with CU Boulder researchers that became the AQ-IQ program.
The current AQ-IQ program brings more than tech into AP Environmental science classes: it also brings CU Boulder engineering students to the classroom. Anna Lisa, the AQ-IQ technical lead and a CU master’s student in Environmental Engineering, describes the college students as mentors who guide high schoolers over the bumps of project design, data analysis, and unexpected results.
Because the high school students brainstorm projects themselves, they aren’t limiting their research to the oil and gas sites near their neighborhoods. Lisa describes a wide range of projects presented at the high school AQ-IQ symposia this March, many of them looking at carbon dioxide (CO2) emissions in everyday life. In one presentation, a group of music students set up a Y-Pod and played loud brass into it, then presented measurements of CO2 output from their horns. Other students placed the pods in their bedrooms at night or in a classroom full of friends to look at CO2 buildup and ventilation. And as reported by the Greeley Tribune, a number of students tested ozone and volatile organic compounds produced by students’ perfumes, fumes from art markers, burning plastic, and manure.
One project tracked ground-level ozone (O3), a pollutant and respiratory irritant when produced at our level of the atmosphere (the troposphere). The student researcher and her family have asthma and allergies, and she wanted to know how she could minimize indoor ozone levels by opening or closing windows at home. She found that since ozone fluctuates throughout the day, opening windows at night or in the early morning minimizes ozone levels inside—as does closing windows during the afternoon when ozone levels are highest. Lisa thought this was one of the more in-depth projects that asked a detailed question and investigated conditions over time.
Exchanging Scholarship
High school students aren’t the only ones learning from AQ-IQ. The program is supported by a year-long class for upper-level Mechanical and Environmental Engineering students at CU Boulder. They take a fall semester crash course in air quality physics, chemistry and environmental science and spend part of the semester performing their own Y-Pod research in order to learn the tech well enough to design and troubleshoot projects with students. During the spring semester, they serve as mentors, visiting the high schools about once a month to teach environmental science, experimental design, data analysis, and research presentation. Maybe most important, they help frustrated students deal with inconclusive results and learn that “negative” data is still useful and valid (something that takes many of us most of grad school to learn).
Other CU Boulder researchers, like Daniel Knight, Lucy Cheadle, and Katya Hafich, have used AQ-IQ as an opportunity to study how project-based approaches impact learning and student attitudes towards science and engineering. Students learned more and became more intrigued by engineering when doing their own research—when they could get the tech to work, that is. When students became frustrated by technical difficulties, they sometimes checked out. The Hannigan lab surveyed high school students and teachers in the AQ-IQ program and used the results to improve the pods. This data contributed to the 2016 upgrade from the earlier U-Pod to the current Y-Pod. The feedback also helped the Hannigan lab improve the instrument’s power supply and develop a data analysis website.
Urban Networking
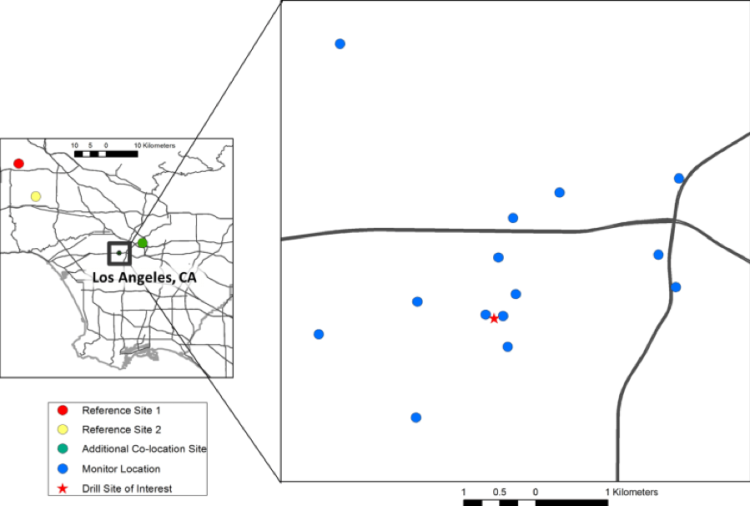
Map of reference sites for data calibration and Y-Pod placement sites for neighborhood data collection near an oil well in Los Angeles. Figure 3 from “Assessing a low-cost methane sensor quantification system for use in complex rural and urban environments.”
Even though the Y-Pods are accessible and affordable, they’re not pared down for education. Their simplicity underlies their value as research tools in communities around the world. The same instrument can study variations in pollutant levels across an urban area just as well as it can measure CO2 blowing through a trombone (though it needs much more careful calibration for the former).
Ashley Collier-Oxandale, an Environmental Engineering doctoral candidate in the Hannigan lab, put her Y-Pods through intense calibration in order to accurately monitor methane (CH4) and other pollutants in two different environments: the Denver metro area, where much of the oil and gas extraction comes from hydraulic fracturing; and Los Angeles, where traditional oil wells are sunk near freeways and residential neighborhoods. Methane doesn’t just hiss and sneak from fossil fuel extraction sites—it can spike. Y-Pods deployed in a network around a facility, throughout a neighborhood, or across a region can pinpoint methane leaks, even though their sensors aren’t as precise as traditional instruments. These networks give researchers much more spatially and temporally detailed information that a single traditional, more expensive, instrument could.
Along the Front Range, Collier-Oxandale and other CU researchers used a grid of Y-Pods to estimate methane emissions across gradients of oil and gas development density and population density. In L.A., she aimed to untangle emissions from neighborhood oil wells from other sources, like traffic.
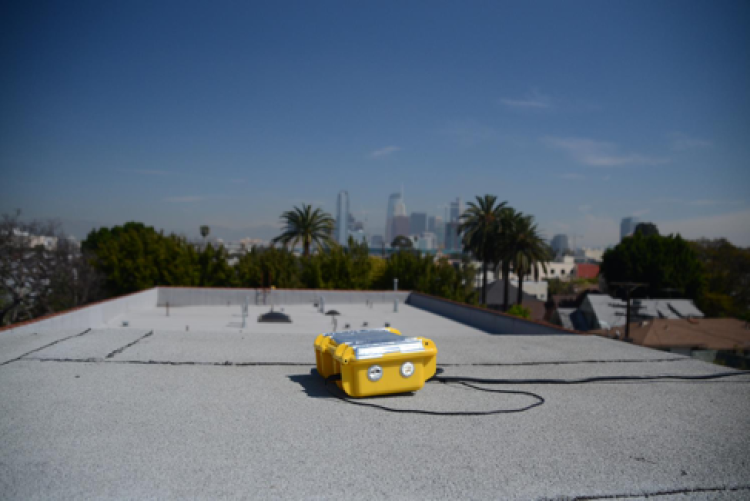
Y-Pods set up around Los Angeles. Photo credit: Jacob Thorson
She worked with housing communities and public health organizers in L.A. to place the Y-Pods in a small cluster near drilling sites and freeways to account for multiple sources of pollution. In addition to hammering out complex models for translating electronic signals into methane concentrations, she outlined the logistics of setting up and maintaining the sensor network with community partners and citizen scientists. Finding a place to set up a Y-Pod depends on identifying an informative spot in the neighborhood with representative air flow and an inhabitant who will let you hike up to the roof or access their property.
“A common response to the question, ‘How good is low-cost sensor data?’ is ‘it depends’”, Collier-Oxandale concludes in her paper about the project. “It depends on what question you are trying to answer, what data you intend to collect, how you would like to use the data, and what supporting measurements are available.”
Air Travels
The Hannigan lab’s community-based research goes beyond developing and deploying the Y-pods in Denver and L.A. In addition to guiding AQ-IQ last year, Lisa traveled to Accra, the capital of Ghana, to train Ghanaian Environmental Protection Agency employees on Y-Pod use, maintenance, and data analysis. Now those researchers are using the Y-Pods for roadside pollutant monitoring.
But Accra has more to worry about than methane belched out by trucks entering the city. Lisa and other CU Boulder researchers have been collaborating with Ghana’s EPA to identify sources of particulate matter pollution in the city. Particles of pollutants, organic molecules, or dust less than 2.5 microns— millionths of a meter, about the same size as a bacterial cell—can penetrate lung tissue, enter the bloodstream, and cause disease. These small particles, also called PM2.5, are generated by cars, manufacturing, waste processing, and wildfires (which have been increasing in size and frequency). Increasing PM2.5 exposure correlates with the risk of cardiovascular disease.
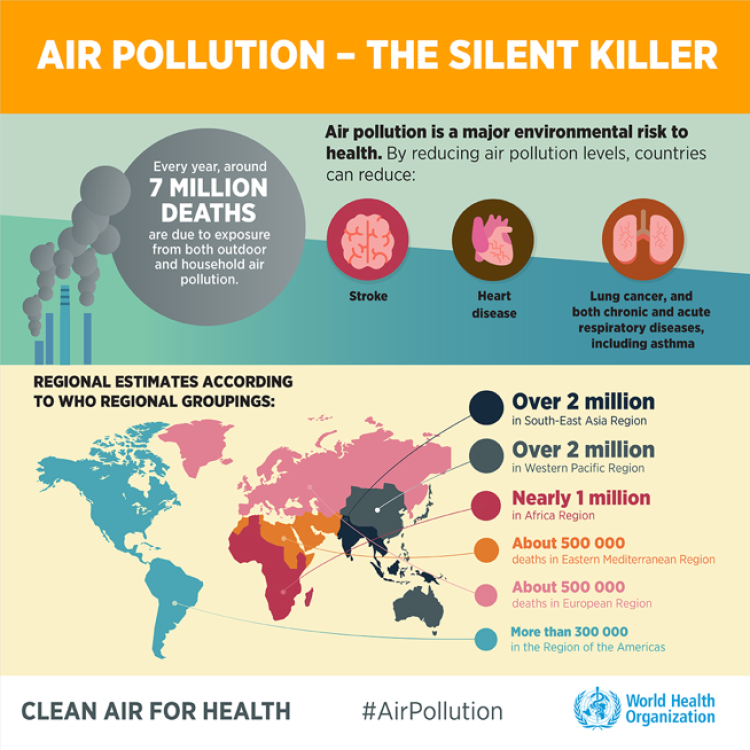
Air pollution is a major cause of stroke, heart disease and respiratory diseases, especially in the developing world. Source: World Health Organization (WHO)
To find the major sources of particulate matter pollution in Accra, Lisa and Hannigan lab Ph.D. student David Pfotenhauer work with the Ghana EPA to trap pollutants by studying filters placed “near a variety of particulate matter sources, including roadside traffic, trash burning, electronic waste burning, [and] cooking” in order to identify “tracers,” molecules that have a unique source emitter.
Ghanaian researchers also collect particulate matter in different neighborhoods. As Pfotenhauer describes, collecting all this data is challenging: “it took us all day to drive around and collect all the samples they had deployed earlier that week. The filter collection sites were scattered all over the city. It was crazy to witness the blank white filters be deployed, and then see them pitch black the next day after 24 hours of sampling the air.” Ghanaian EPA and Boulder researchers use a chemical analysis technique called gas chromatography/mass spectrometry (GC/MS) to identify the precise concentrations of compounds stuck to each particle-clogged filter. They plan to compare compound concentrations from known pollution sources to data from each neighborhood to identify tracers and determine what combination of sources contributes to particulate matter pollution across the city. Creating that complex Venn diagram involves some tricky chemistry, math, and programming.
Pfotenhauer describes tricky conditions, too. “The scientists working in the lab are brilliant and very excited about the work they’re doing – but often the lack of equipment or funding can bring a sudden halt to projects or experiments.” But despite those limitations, the Ghanaian researchers keep going, “holding themselves accountable in regards to human health, global energy use, and hazardous emissions. I really hope that more collaborations like this start forming all around the world – such that any country or organization that wishes to start thinking about sustainability and protecting our world’s environment can do so.”
After talking to Lisa about the breadth of projects happening in her lab, one thing stuck with me. “So many people need the resources developed by engineers”, she said and emphasized that engineering isn’t just about designing the next iPhone. Engineers can solve urgent problems. Respiratory diseases are among the biggest causes of death and disability in developing countries. The WHO estimates that 7 million people die prematurely each year due to air pollution, a statistic that pulled Lisa into her current work. In the US, air quality research contributes to public environmental and health policy.
That quest for public solutions takes many forms: developing inexpensive instruments, teaching high school students about science, and venturing out into new communities. Citizen engineering goes beyond teaching and beyond sample collection. It can involve helping a high school student rev up a car battery, recording neighborhood air quality observations, or collecting soot-stained filters. It means training researchers from Greeley to Ghana to spark new projects. It means asking someone to let you (and your research) onto the roof.
This article was originally published by Bridget Menasche on August 23, 2018 as part of the Science Buffs STEM Blog.