Research
Research Projects
Our lab centers on interdisciplinary research at the frontiers of Biology, Medicine, Physics, and Micro/Nano Engineering. We focus on creating cutting-edge micro/nano systems for various applications in life science, healthcare, and medicine. Current research projects include:
- Biomedical devices for next generation precise drug delivery. Intracellular delivery are required in diverse scenarios ranging from cell-based immunotherapy and gene editing to regenerative medicine and fundamental biology. We are developing a next generation drug delivery platform by confining the perturbation energy on the surface to precisely control the cell membrane permeability, thus the delivery dose at an unprecedented level. This NanoEngineered Surface Technology (NEST) based drug delivery system can be integrated into a standard pipette tip to change the way of current drug delivery style. We aim to first apply this technology for T cell based immunotherapy.
- Microfluidic device for cell mechanics: Biomarkers, or biological markers, are measurable indicators of biological state or condition. The measurement and characterization of mechanical biomarkers (mechanical properties) of cells, such as mass, compressibility, viscosity, stiffness, and density, has been of great interest to biomedical researchers and could have profound impacts in cellular biology, cancer, disease, and drug research. We are developing an acoustic microfluidic device to simultaneously measure multiple mechanical biomarkers of individual cells with a super-high throughput. The measurement of multiple-dimension mechanical biomarkers of individual cells in high throughput is beyond the capability of any current technology and could provide an entirely new foundation for both fundamental cell biology and clinical research.
- On-chip cell/molecule handling and analysis. We are developing Lab-on-a-Chip devices that can precisely manipulate cells, molecules and fluid chemical species for sample preparation and analysis. Particularly we integrate multiple acoustic functions, including, acoustic manipulation/separation/patterning, acoustic streaming and mixing, acoustic sensing, and acoustic heating, into a single system to combine sample preparation and concentration, cell lysis, and data collection, to simplify all the processes and achieve comprehensive functions for fast diagnosis. It will generate high impact to life science and clinical applications.
- On-chip C elegans handling and analysis. C elegans is a model organism in biological studies. We are developing Lab-on-a-Chip tools that can precisely manipulate such little animals to provide a quantitative analysis which is challenging by current methods.
We heartfully appreciate funding support from CU Boulder, W.M. Keck Foundation, NIH NIGMS, NSF, etc. for their generous support to our research.
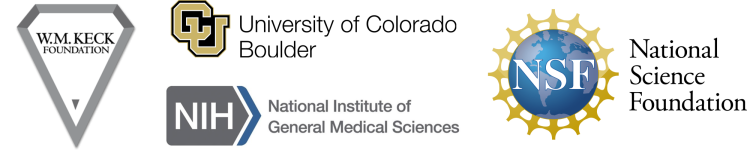
Research Achievement
On‐Chip Acoustic Manipulation for Protein Analysis

Yonghui Ding, Kerri A. Ball, Kristofor J. Webb, Yu Gao, Angelo D'Alessandro, William M. Old, Michael HB Stowell, and Xiaoyun Ding. "On‐Chip Acousto Thermal Shift Assay for Rapid and Sensitive Assessment of Protein Thermodynamic Stability." Small 16, no. 41 (2020): 2003506.
Thermal shift assays (TSAs) have been extensively used to study thermodynamics of proteins and provide an efficient means to assess protein–ligand binding or protein–protein interactions. However, existing TSAs have limitations, such as being time consuming, labor intensive, or having low sensitivity. Herein, an acousto thermal shift assay (ATSA), the first ultrasound enabled TSA, is reported for real‐time analysis of protein thermodynamic stability. It capitalizes the coupling of unique acoustic mechanisms to achieve protein unfolding, concentration, and measurement on a single microfluidic chip within minutes. Compared to conventional TSA methods, the ATSA technique enables ultrafast (at least 30 times faster), highly sensitive (7–34 folds higher), and label‐free monitoring of protein–ligand interactions and protein stability. ATSA paves new avenues for protein analysis in biology, medicine, and fast diagnosis.
Microfluidics for intracellular/subcellular drug delivery in high throughput

1. Xiaoyun Ding, Martin P Stewart, Armon Sharei, James Weaver, Robert Langer, Klavs F Jensen, High-throughput nuclear delivery and rapid expression of DNA via mechanical and electrical cell-membrane disruption. Nature Biomedical Engineering, 0039, (2017).
2. Martin P Stewart, Armon Sharei, Xiaoyun Ding, Gaurav Sahay, Robert Langer, Klavs F Jensen, In vitro and ex vivo strategies for intracellular delivery. Nature 538 (7624), 183-192 (2016)
Nuclear transfection of DNA into mammalian cells is challenging yet critical for many biological and medical studies. Here, by combining cell squeezing and electric-field-driven transport in a device that integrates microfluidic channels with constrictions and microelectrodes, we demonstrate nuclear delivery of plasmid DNA within 1 hour after treatment, the most rapid DNA expression in a high-throughput setting (up to millions of cells per minute per device). Passing cells at high speed through microfluidic constrictions smaller than the cell diameter mechanically disrupts the cell membrane, allowing a subsequent electric field to further disrupt the nuclear envelope and drive DNA molecules into the cytoplasm and nucleus. By tracking the localization of the ESCRT-III (endosomal sorting complexes required for transport) protein CHMP4B, we show that the integrity of the nuclear envelope is recovered within 15 minutes of treatment. We also provide insight into subcellular delivery by comparing the performance of the disruption-and-field-enhanced method with those of conventional chemical, electroporation, and manual-injection systems.
Cancer Cell Separation
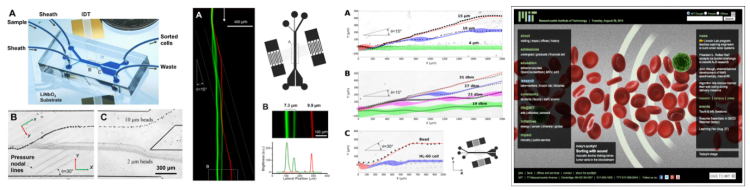
Xiaoyun Ding, et al., "Cell separation using tilted-angle standing surface acoustic waves" Proceedings of the National Academy of Sciences of the United States of America (PNAS), 111, 12992-12997 (2014).
(Highlighted as a News Report onMIT Home Page. Carnegie Mellon News. Daily News. Breast Cancer News Today. Headlines and Global News. The Daily Dot. Medical News Today. Science 2.0. e-Science News. Laboratory Equipment. R&D. Phys Org. Gizmag. Quantum Times. Nanowerk. Science Daily. Daily News. Technobahn. Open Nano. Jersey Tribune. Penn State News.)
We have developed a unique approach for the separation of particles and biological cells through standing surface acoustic waves oriented at an optimum angle to flow direction of cells and particles in a microfluidic device. This experimental set-up, optimized by systematic analyses, has been used to demonstrate effective separation based on size, compressibility, and mechanical properties of particles and cells. The potential of this method for biological/biomedical applications was demonstrated through the example of isolating MCF-7 breast cancer cells from white blood cells. The method offers a possible route for label-free particle or cell separation for many applications in research, disease diagnosis and drug-efficacy assessment.
Standing SAW based Acoustic Tweezers
Xiaoyun Ding, et al., PNAS, Vol. 109, pp. 11105-11109, 2012. (was reported and highlighted by Nature Methods, National Science Foundation, National Institutes of HealthLive Science, Big Ten Science, Lab Spaces, One News Page, gantdaily, MSNBC, R&D, Medical News Today, Nanowerk, Science Daily ,Popular Science, Online Journal, Newswise, domain-b, Bio-Medicine, Penn State Live, Penn State Science, Real Clear Science, Sci Tech Update, Phys Org, UPI.com, Softpedia, ALN Magazine, SciTechDaily, Futurity, DVICE, Inside Science, )
We have developed the first acoustic manipulation platform, so-called “acoustic tweezers”, which can trap and dexterously manipulate single microparticles, cells, and entire organisms (i.e., Caenorhabditis elegans) along a programmed route in two-dimensions within a dime-sized microfluidic chip. Fig. 1 A shows the real photo of our acoustic tweezers device. The acoustic tweezers can move a 10-µm single polystyrene bead to write the word “PNAS” (fig.1 B) and a bovine red blood cell to trace the letters “PSU” (fig. 1 C). It was also the first technology capable of touchless trapping and manipulating Caenorhabditis elegans, a one-millimeter long roundworm that is an important model system for studying diseases and development in humans.With its advantages in non-invasiveness, miniaturization, and versatility, our acoustic tweezers will become a powerful tool for many disciplines of science and engineering.
Acoustic tweezers based multichannel cells sorting
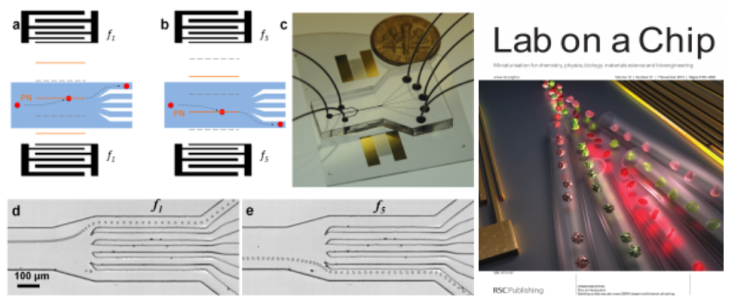
Xiaoyun Ding, et al., Standing Surface Acoustic Wave (SSAW) Based Multichannel Cell Sorting, Lab on a Chip, Vol.12, pp. 4228–4231, 2012. (was highlighted and reported by Kurzweil, NSF, Human Health & Science, Quantum Times, Futurity, SIP TRUNKING, DeviceSpace, EurekAlert, UPI, e-Science News, LabSpaces, Health Canal,TG Daily, Gizmag, PhysOrg, Product Design and Development, Science Daily, Laboratory Equipment, Azonano, The Engineer, R&D, )
Cell sorting is essential for many fundamental cell studies, cancer research, clinical medicine, and transplantation immunology. We developed an acoustic-based method that can precisely sort cell into five separate outlets of cells (Figure to the right), rendering it particularly desirable for multi-type cell sorting. Our device requires small sample volumes (~100 μl), making it an ideal tool for research labs and point-of-care diagnostics. Furthermore, it can be conveniently integrated with a small power supply, a fluorescent detection module, and a high-speed electrical feedback module to function as a fully integrated, portable, inexpensive, multi-color, miniature fluorescence-activated cell sorting (μFACS) system.
Tunnable cells patterning
[Xiaoyun Ding, et al., Lab on a Chip, Vol. 12, pp. 2491-2497, 2012. (featured as back cover image) ]
The ordered arrangement of biological cells and microscale objects plays an important role in many biological and colloidal studies such as microarrays, regenerative medicine, and tissue engineering. We have developed an acoustic-based tunable patterning technique that can control the cell pattern with the tunability of up to 72% [2]. This acoustic-based tunable patterning technique has the advantages of wide tunability, non-invasiveness, and ease of integration to lab-on-a-chip systems, and shall be valuable in many biological and tissue endingeering studies such as investigation of cell-cell interactions. Due to its significance, this work was selected as cover image at the journal Lab on a Chip.
SAW driven light shutter using polymer-dispersed liquid crystals (PDLCs)
A surface acoustic wave (SAW)-driven light shutter is demonstrated using polymer-dispersed liquid crystals (PDLCs). Our experiments show that a PDLC film switches from a non-transparent state to a transparent state when subjected to a SAW. The working mechanism is analyzed theoretically and the acousto-optic properties of the PDLC film are characterized. This switching behavior results from the acoustic streaming-induced realignment of liquid crystal (LC) molecules as well as SAW-induced thermal diffusion. Such a device shows an excellent performance in terms of imaging quality and optical contrast, which is important for display applications and smart windows.