Research
Computer simulations of molecular phenomena are increasingly successful aids to chemical engineering, bioengineering, and materials science research. Simulation is particularly suited for providing all-atom insight that is inaccessible through experiment and for investigating large numbers of chemical entities and conditions that are expensive or difficult to physically create. As computer power continues to increase exponentially, atomistic simulation will become even more useful.
In the Shirts group, we are working on the improved simulation methods necessary to make molecular simulation a vital part of the chemical engineering toolkit for designing new materials and making accurate thermodynamic property predictions. We use these advances to perform challenging or previously impossible molecular design in the context of drug discovery, protein processing, and materials design, and collaborate with experimentalists to test molecular hypotheses inaccessible through direct experiment. Our eventual goal is to enable design of both biological and nonbiological molecular machinery that can improve human health and standards of living in a sustainable way.
Further descriptions of some of our main research projects are below:
Improving computer-aided drug design
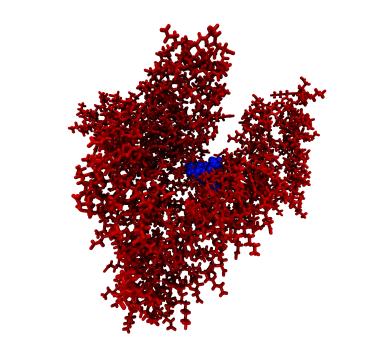
Drug resistance is one of the biggest challenges in the pharmacological treatment of infectious diseases, and current informatics based drug discovery methods are not well suited to rapidly develop new drug variants that can successfully overcome resistance. Our research has demonstrated that statistical mechanical methods can predict ligand binding affinities to within 1 kcal/mol in simple atomistically detailed systems, a level that becomes useful for the pharmaceutical industry. However, significant effort is necessary to make such methods work in more typical drug systems and to make them scale efficiently enough to be useful in general practice. We are using molecular simulation techniques to make these calculations, faster, easier, and more reliable, and to explore problems such as binding of pharmaceuticals to human serum proteins.
Improving molecular simulation and computational property prediction
The most pressing problem in the atomic-level simulation of polymers, macromolecules, and other complicated dense fluids is the lack of sufficient sampling to accurately measure and observe physical phenomena. Without sufficient conformational sampling, it is impossible even to verify if models are sufficiently faithful to experiment, let alone explore behavior of either long time scales or of larger molecular systems. It is currently only possible to simulate the equivalent of a few microseconds of all but the smallest biological systems, with some heroically expensive extensions to milliseconds with large supercomputers. In the Shirts group, we have made important contributions to efficient analysis of free energy calculations and other thermodynamic data; our current research focuses on methods for sampling both chemical and configuration space of heteropolymers and complex fluids. We are actively involved in the Open Force Field Initiative, an open science and open data effort ot develop improved atomistic force fields.
Understanding and predicting thermodynamics of crystalline solids
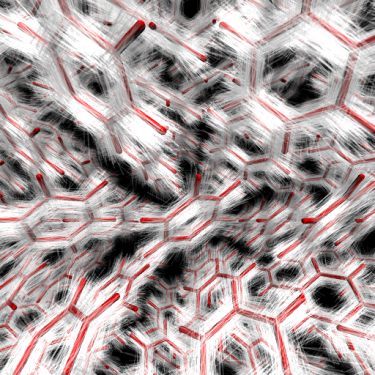
Much of our understanding of solids uses approaches that to a large extent assume zero temperature crystal minima and harmonic approximations around these minima. In realistic systems, such as crystals of pharmaceutical agents or metal alloys and oxides used in structural or functional materials, we need to know what is happening at ambient conditions or even hotter temperatures, where these approximations break down. The Shirts group is bringing together methods to achieve the necessary levels of sampling required to understand where realistic crystal behavior diverges from simple theories, and to understand exactly how detailed our models of these systems need to be to make useful predictions that can guide pharmaceutical processing engineers and materials scientists. We are using both molecular simulation and machine learning to improve our abilities to predict thermodynamics and properties.
Designing improved purification materials
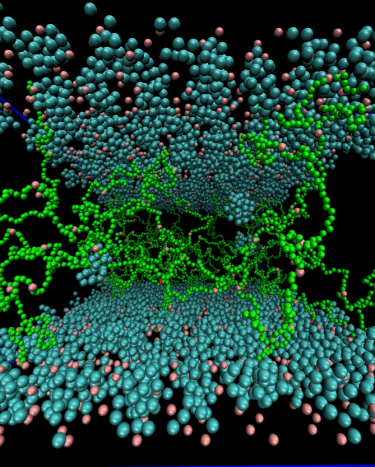
Production of clean water is one of the most important engineering challenges of the 21st century, but the energies involved in separating out salts and other contaminants are frequently cost-prohibitive. The production of organic chemicals and fuels via biotechnology generally requires separation of organic starting materials, product molecules, and reaction byproducts from aqueous solution, as well as the removal of water from the desired species. We are working on designing and understanding membranes for improved separations of ions and small molecules using molecular modeling of the most basic transport and sorption properties of novel polymeric materials.
Designing novel heteropolymeric materials
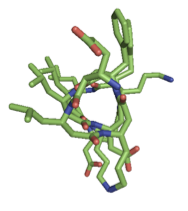
The wide physical and chemical diversity of biomolecular processes strongly suggests that the possibilities for novel function in human-engineered materials are far, far beyond our current capabilities. Designed materials can draw from a much larger range of chemical structure and functionality than exists biologically; if we can add significant chemical diversity to nature's already impressive toolkit, what else can be created?