Cuk Research Group isolates reaction step that describes energetics of catalysis on materials
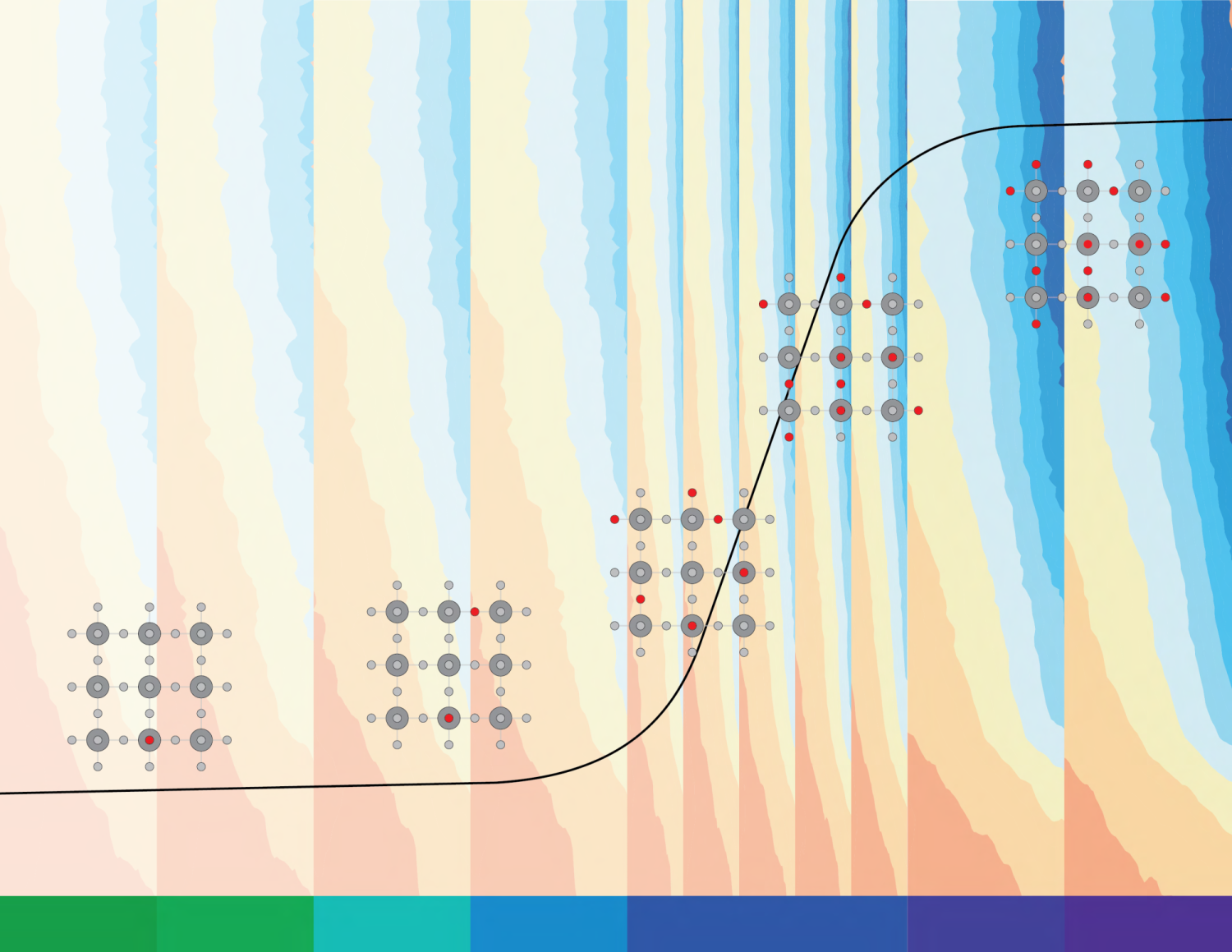
Associate Professor Tanja Cuk
New research published in Nature Materials from Associate Professor Tanja Cuk and colleagues sheds light on a fundamental chemical reaction — the breaking apart of water to produce a molecular fuel such as hydrogen. Cuk is faculty in the Department of Chemistry and the Materials Science and Engineering Program (MSE) and is a Fellow in the Renewable and Sustainable Energy Institute (RASEI).
When water undergoes such a reaction, there are a number of discrete reaction steps that should occur in sequence. Cuk’s lab specializes in timing these steps and therefore isolating individual reactions as part of the broader process.
“This paper isolated one of the reaction steps in time, so we can then learn more about the energetics of that discrete step — how strong or weak the bonds are before and after it — within the broader reaction,” Cuk said.
Understanding the free energy needed to form a chemical is key to its usefulness as a fuel source. If an element has weak electrochemical bonds, it has a high-energy potential, because it can be broken apart easily.
“Similar to what plants do naturally, we are trying to break the bonds of water and reform the bonds of oxygen,” Cuk said. “The protons left over create hydrogen for fuel.”
Cuk and her group used a pulsing photovoltaic effect to generate current and a tuning of the water’s pH to get more products of the first step — reaction intermediates — with higher pH. This creates a curve — the reaction isotherm of Figure 4 in the manuscript and depicted in Figure 1, right — from which the free energy change of that reaction step can be determined.
Figure 1. The time-resolved optical data as a function of pH (litmus scale on the bottom) show how the population of intermediates generates a reaction isotherm (black trace). The emission (in blue) counts the intermediate population (cartooned) and from its growth, one obtains the free energy change for the first electron transfer from water. Figure constructed with the aid of Daniel Morton of RASEI.
“The electrochemical reaction was driven by the charge,” Cuk said. “We have an electrode and we shine light on it. That is the energy inputted into the whole reaction. But we shine pulsed light, so we can time the separate reaction steps within the total fuel-producing reaction.”
Because sunlight is continuous, it can obscure the individual steps associated with a chemical reaction. Using pulsed light in principle allows the researchers to experimentally determine the free energy needed for each meta-stable reaction step. However, a pulsed light spectroscopy, usually called pump-probe or time-resolved spectroscopy, had yet to live up to this.
This work experimentally determined the free energy change of the first electron and proton transfer from water at a material surface. This understanding is especially important for materials science because theorists use it to differentiate the activity of water splitting catalysts. If this is a difficult, energy-intensive process, the catalyst is not as efficient at producing the final fuel, hydrogen.
Ilya Vinogradov, a postdoctoral researcher in the Cuk Research Group and with RASEI, contributed to building the transient reflectance setup, wrote new data acquisition software and mentored the graduate students in data acquisition and analysis. MSE graduate students Suryansh Suryansh and Hanna Lyle acquired the data and processed the data sets. Michael Paolino, a graduate student in Physics, aided in the latest data collection. Vinogradov furthered the SVD analysis spearheaded by Research Associate Aritra Mandal and contributed to the rotation analysis described in Figure 2.
“This research is impactful because it provides an experimental measure of an important theoretical materials performance predictor — free energy change of the first electron transfer step — for water splitting catalysis,” Vinogradov said. “If a material’s descriptor correlates well with device performance, one can computationally optimize its chemical composition and crystal structure for the descriptor in lieu of directly measuring the device’s performance.”
This allows researchers to evaluate different materials as high performers more efficiently and quickly, saving time and cost for materials that would otherwise need to be subject to experimentation.
“Although our method is not quite there yet in terms of throughput and precision, one way a more solid experimental footing may help improve the materials design strategy is by discovering non-idealities that the theorists may have missed,” Vinogradov said. “This can help improve the descriptor's predictive accuracy.”
Cuk said some of these non-idealities would have to do with the reaction kinetics, or the timing with which the intermediate products turn over, rather than directly with the free energies of each reaction step.
“This is important for a truly sustainable energy storage cycle, where the rate at which products turnover keeps up with the rate at which rays of sunlight come in,” she said. “By using a time-resolved spectroscopy, we can get at both the free energies and the rates that circumscribe fuel production.”