Scientists construct first-ever synthetic DNA-like polymer
These synthesized helical covalent polymers represent a huge advance for this critical and understudied field, researchers say
Double helical covalent polymers—which are spiraling collections of nature’s building blocks—are fundamental to life itself, and yet, despite decades of research, scientists have never been able to synthesize them in their entirety like their non-helical brethren—until now.
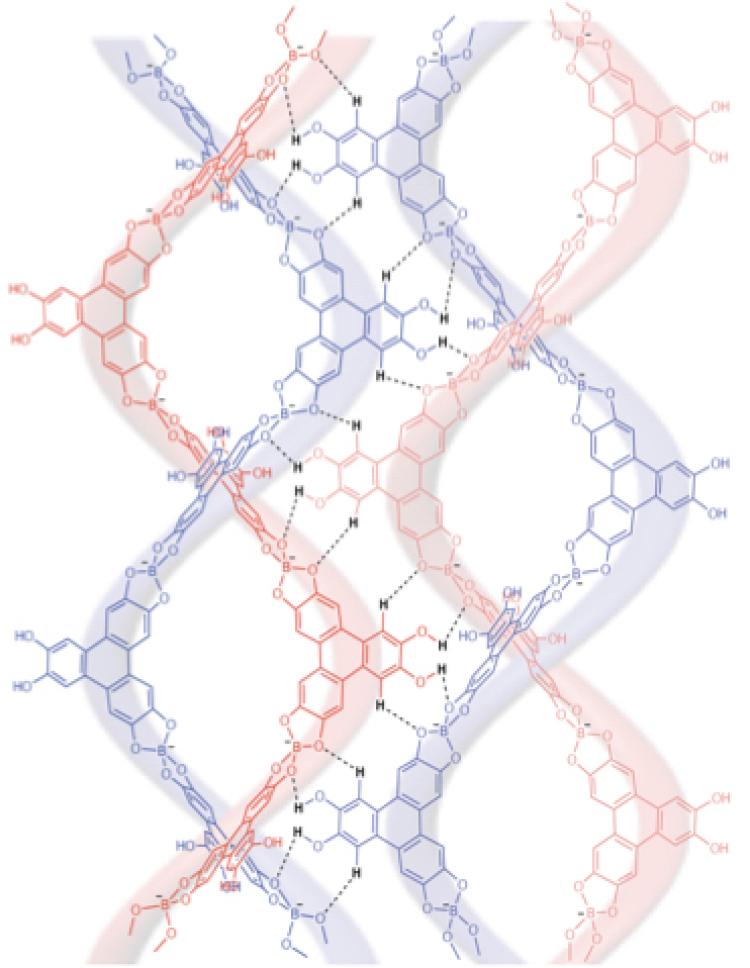
Formation of pairs of entwined helical strands through an array of hydrogen-bonding interactions along the a axis. The closest interstrand distance within one pair was measured from Ar–H to H–Ar (6.5 Å).
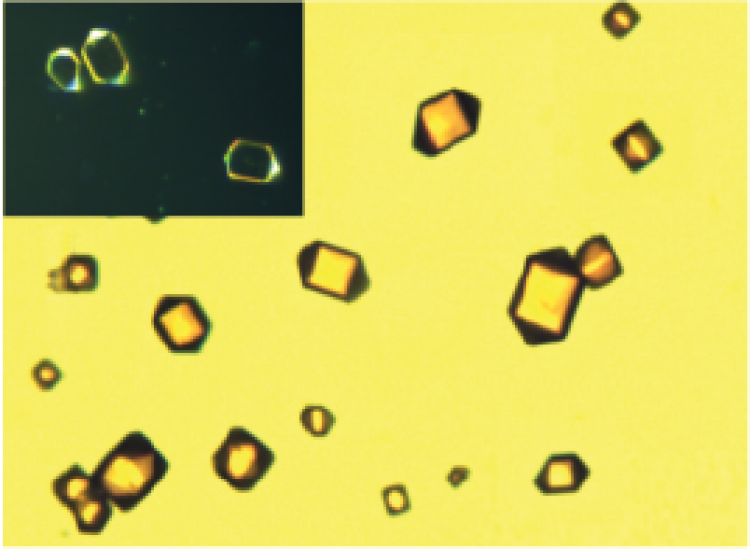
Optical images of the large single crystals of 1. 1 grew in elongated square bipyramid shapes. Inset: dark-field optical microscope image with high contrast at the edges.
Scientists, led by a team at the University of Colorado Boulder, have cracked the code, creating synthetic versions of these large DNA-like molecules for the first time. Using dynamic covalent chemistry, which is a chemistry tool pioneered by these researchers that focuses on reversible bonding interactions with self-correction capabilities, they were able to not only construct a helical covalent polymer that rivals the sophistication of those found in nature but confirm its existence with absolute certainty using single crystal x-ray diffraction (a powerful, non-destructive way to characterize single crystals using light).
Previously, scientists have only been able to solve individual parts of the puzzle. This new discovery out last week in Nature Chemistry, though, completes it, potentially opening this critical and understudied field to new research that could have implications on everything from artificial enzyme creation, which has already found success in various medical applications, to the creation of biomimetic materials (materials that mimic processes found in nature).
“People very rarely can see what’s really going on in synthetic polymers in terms of atom spatial locations, inter-chain interactions, how they’re bonding, how they twine and wind at the atomic level,” said Wei Zhang, an author on the study and a professor of chemistry at CU Boulder. “With single crystals, though, we can really experimentally visualize the atom, the bonds, how long it is, how they interact. That’s why getting the single crystal structure of a polymer is a very, very big deal.”
Polymers are substances or materials formed by the buildup of lots of smaller, similar units (like glucose and amino acids) bonding together either naturally or synthetically. Naturally occurring polymers can include silk, wool, DNA, proteins, enzymes and cellulose, whereas synthetic polymers are manufactured by either scientists or engineers and include materials like plastics.
Synthetic polymers come in many forms depending on their construction—whether they are linear or helical, the number of strands, and the length of the strands. Of those, helical polymers have been the most challenging for scientists to synthetically replicate, with the double stranded being the most difficult of all, thus far limited to only short helical oligomers (a polymer with very few repeating units).
That is, until this new research.
Zhang and colleagues were able to use a chemical tool they’ve pioneered, dynamic covalent chemistry, to construct a DNA-like covalent helical polymer. When they did that, the large molecule wasn’t the only thing they discovered.
They also found single crystals.
“That came as a nice surprise,” commented Zhang. “At the end of the reaction when we noticed there were some shining single crystals lying at the bottom of the reaction vessel, we were thrilled. We said, ‘Wow! Okay, let’s give it (x-ray diffraction) a shot.’ Getting a single crystal of a polymer is extremely rare.”
Using single-crystal synchrotron X-ray diffraction, the researchers were able to confirm, without a doubt, that they had created what had previously been impossible.
This discovery, though, is only the beginning both for them and this critical field of study.
After they dive a little deeper into the structure itself, the researchers plan to play with and explore the structure itself, seeing if they can make the crystals themselves bigger (right now they are fairly small), and if they can control the chirality, or spiral nature, of the polymer, which could have broad implications for catalysis (chemical reaction process utilizing catalysts), signal transduction (how signals are sent throughout the cell) and sensing applications.
“There is a lot of rational design, synthesis, structure-property relationship work that we need to do,” Zhang said. “Ultimately we want to demonstrate this is a very powerful platform for smart biomimetic materials design.”
Other authors on this paper include Yiming Hu, Yinghua Jin, Hongxuan Chen, Jingyi Wu from CU Boulder; Simon J. Teat from the Lawrence Berkeley National Laboratory; Wei Gong and Yong Cui from Shangai Jiao Tong University; Zhou Zhou, Tao Jiang and Xinbin Cheng from Tongji University.