Interaction of an expanding plasma plume with ambient gas in pulsed laser deposition
Background:
Pulsed laser deposition (PLD) involves a laser ablation procedure, where a laser pulse interacts with a material surface to induce the formation of a plasma plume that expands away from the surface. This plume contains material that is eventually deposited on an opposing substrate similar to previous deposition processes studied in NGPDL. The interaction of the plume with a background gas influences the efficiency of the deposition process and is associated with a myriad of accompanying physical mechanisms. For example, a plume expanding into ambient gas of low pressure around 100 mTorr exhibits plume splitting and sharpening [1]. A definitive mechanistic explanation for laser-induced plasma plume splitting remains an active area of research [2]. The splitting of the plume into fast and slow components influences the geometry of the plume and can lead to effects on deposition characteristics that may be of industrial relevance.
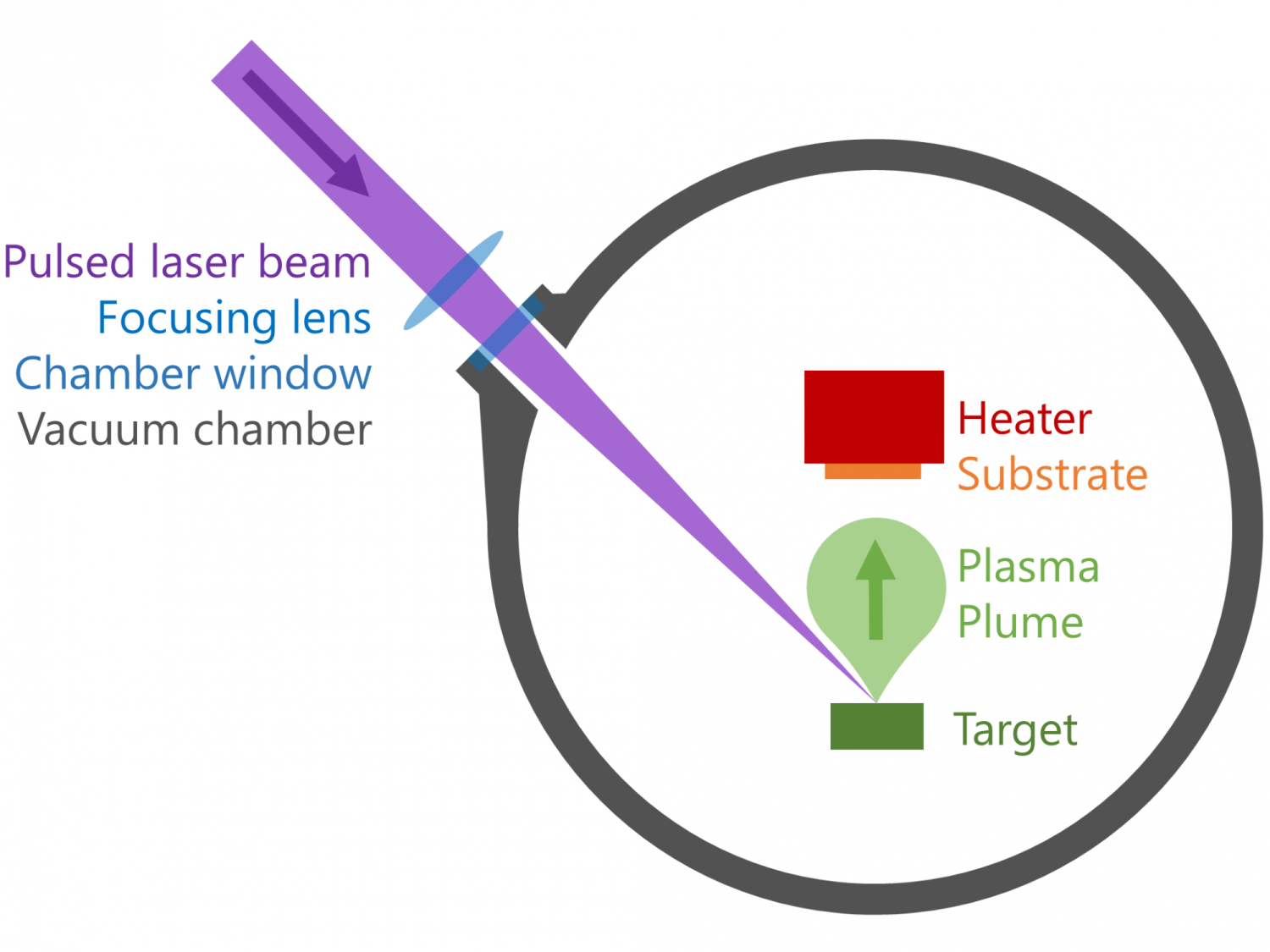
Figure 1. Schematic of PLD. (Image credit: Tedsanders, Wikimedia Commons, CC0 1.0)
Methods:
Plasma behavior may be simulated using particle methods such as particle-in-cell (PIC) and direct simulation Monte Carlo (DSMC), or Eulerian methods such as the direct kinetic (DK) method that directly solves the Boltzmann or Vlasov equation. Both methods have been previously studied in NGPDL. The DK method does not suffer from the statistical noise inherent to particle methods and is suitable for time-varying problems. In addition, it is able to effectively simulate rarefied regions where particle methods may have sparse populations depending on the initial and flow conditions. In PLD, the plume of interest expands unsteadily into an initially rarefied region, making the DK method well suited to the problem.
Results:
The development of a DK method for two atomic species with their charged counterparts is underway. A DK method for two neutral atomic species with cross collisions has been developed as an intermediary step to this goal. The following plots reveal the nature of these cross collisions.
Figure 2(a) plots the initial atomic number densities in a two-species population residing in a one-dimensional domain, while Figure 2(b) plots the corresponding number densities after some time has elapsed. The dense plume diffuses and expands to the right, while some of the background gas is also accelerated to the right due to cross collisions.
(a)
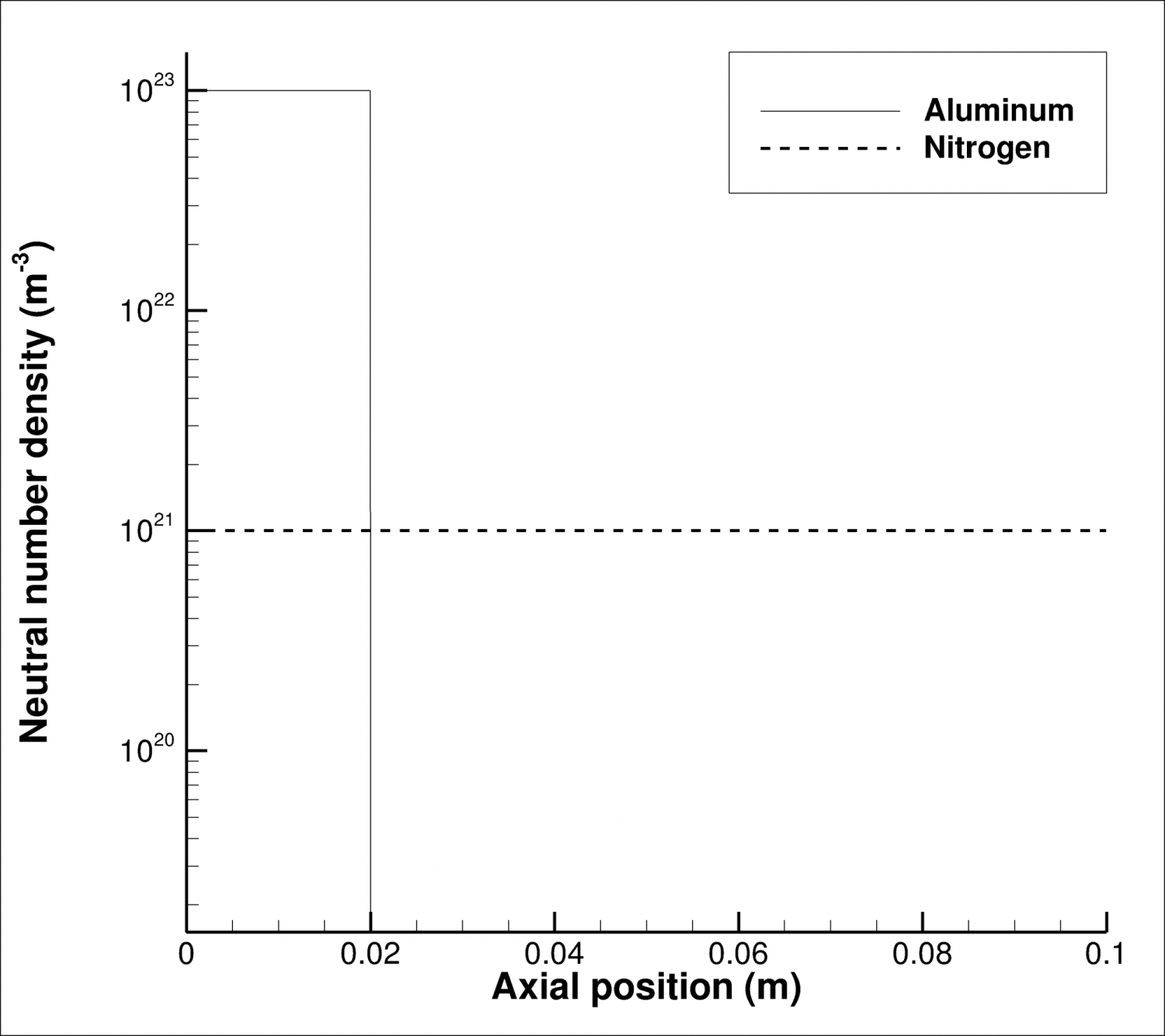
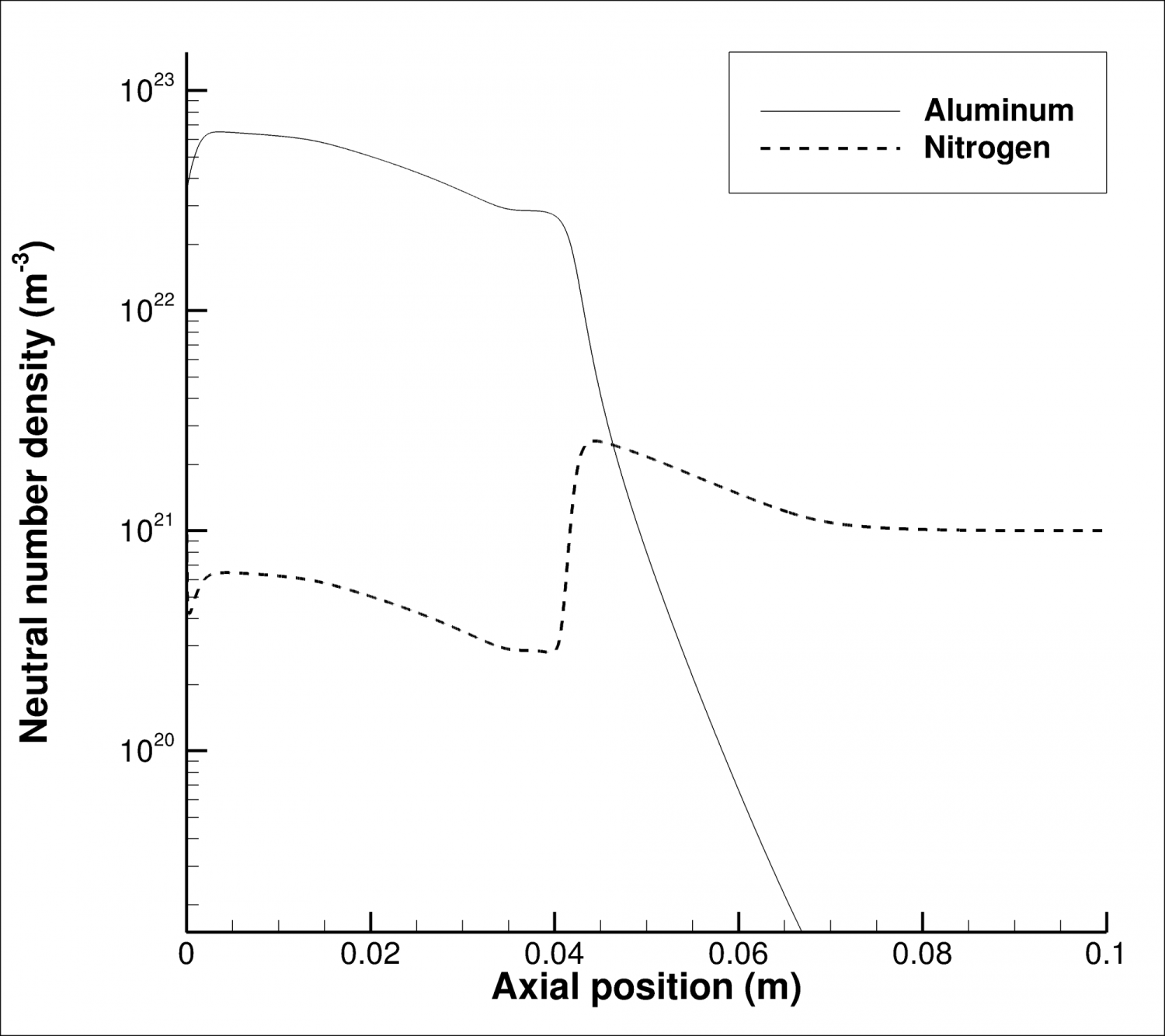
Figure 2. (a) Initial atomic number densities in a two-species population as functions of space. (b) Corresponding number densities after some time has elapsed.
As a verification exercise for the cross-collisions routine, three setups with identical total number densities were initialized and allowed to evolve in time. Their total number densities after some time has elapsed are plotted in Figure 3. The single-species configuration and the two-species configuration with cross collisions agree to a reasonable extent as expected, while the total number density in the two-species configuration without cross collisions diffuses more quickly with fewer collisions to inhibit the expansion process.
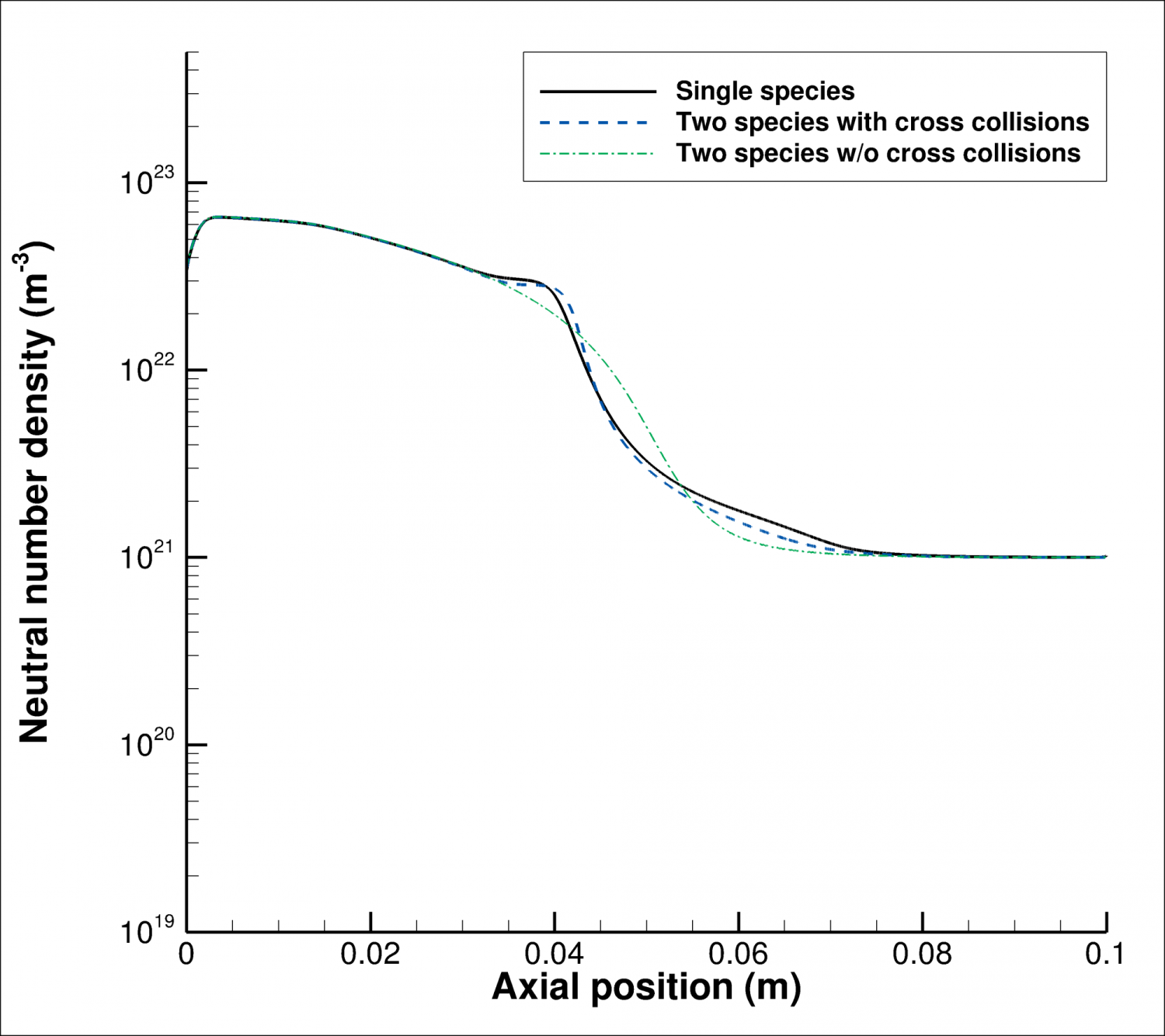
Figure 3. Comparison of total number densities in a single-species population, a two-species population with cross collisions, and a two-species population without cross collisions, at the same time instance as in Figure 2(b). Here, the two-species configurations include two identical constituents for an apples-to-apples comparison with the single-species configuration.
Investigators:
References:
[1] S. S. Harilal, C. V. Bindhu, M. S. Tillack, F. Najmabadi and A. C. Gaeris, “Internal structure and expansion dynamics of laser ablation plumes into ambient gases,” Journal of Applied Physics, Vol. 93, pp. 2380–2388, 2003.
[2] H. Yuan, A. B. Gojani, I. B. Gornushkin, X. Wang, D. Liu and M. Rong, “Dynamics of laser-induced plasma splitting,” Optics and Lasers in Engineering, Vol. 124, 105832, 2020.