Hypersonics
As illustrated in February 2021 by NASA’s landing of Perseverance on Mars, one of the most dangerous and challenging aspects of any planetary exploration mission is a spacecraft's hypersonic entry into a planet's atmosphere. Achieving this goal has involved many succesful missions over the past years. The physical processes occurring around the spacecraft are quite complex and involve the synthesis of chemical kinetics, quantum mechanics, radiation physics, and ablation effects with fluid dynamics. To further complicate matters, the atmosphere is often rarefied and conventional fluid dynamic analysis is no longer applicable. Such high energy, high speed, rarefied conditions are very expensive and often impossible to reproduce in wind tunnels here on Earth. Actual flight tests are even more expensive and measured data is limited to that collected during the 1960's Mercury, Gemini, and Apollo programs. If numerical simulation can reproduce the experimental data that is available, it can then be used with confidence as a fast and inexpensive design tool for new spacecraft flying new missions.
At low altitudes (below ~80km for Earth) the atmosphere is sufficiently dense such that molecules undergo a vast number of collisions as they move over the spacecraft. Under these conditions the gas can accurately be assumed to behave as a continuum and the Navier-Stokes equations can be solved using methods from Computational Fluid Dynamics (CFD). CFD methods are very mature and are capable of incorporating advanced physical models such as chemical and thermal non-equilibrium, radiation, and even ablation. For very high altitudes (above ~100km for Earth) the atmosphere is rarefied to the point where molecules undergo far fewer collisions invalidating the continuum assumptions inherent in the Navier-Stokes equations. In this regime the most mature numerical method is the direct simulation Monte Carlo (DSMC) method which is also capable of incorporating advanced physical models. Since the DSMC method simulates the gas on the molecular scale it provides accurate results in all regimes, however under continuum conditions, large numbers of particles and collisions demand impractical computational resources. Thus, in general, the DSMC method is used to simulate atmospheric entry at high altitudes and CFD is used at lower altitudes. Of course there is a large overlap regime in which the flow around the spacecraft exhibits regions of both continuum flow and non-equilibrium or rarefied flow. For this reason current research is not only focused on using CFD and DSMC to simulate the aerothermodynamics of atmospheric entry, but also focuses on incorporating these methods into a hybrid particle-continuum code.
Introduction
At the high temperatures encountered by hypersonic vehicles, gasses chemically react. Room temperature air is mostly N2 and O2, but around 3000 K oxygen starts to dissociate, or break down into its constitutent atoms. Nitrogen, which has a stronger bond, dissociates starting around 6000 K. These processes have a profound effect on the gas environment surrounding hypersonic vehicle, for several reasons. First, dissociating a molecule takes energy, and so the gas cools as it dissociates, decreasing the heating experienced by vehicles in flight. Second, breaking one molecule into two atoms increases the pressure, potentially affecting the shock shape and vehicle aerodynamics. Finally, different chemicals interact differently with surfaces; atomic oxygen in particular is highly reactive with carbon-based heat shields (see also the section on Material Response & Ablation). Therefore, we must be able to predict the chemical evolution of a gas at high temperatures.
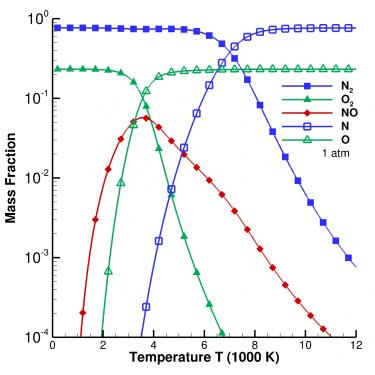
Mass fractions of air at chemical equilibrium for 1 atmosphere, neglecting ionization
Nonequilibrium Chemical Kinetics
A hot gas dissociates very quickly, on the order of microseconds. One might think we could neglect the speed of dissociation, then, and model the gas in an equilibrium state. However, many things happen very quickly in hypersonic flows, and so the speed of reaction often matters. For example, suppose that the air behind a strong shock is very hot and travelling at 1 km/s. If dissociation takes 10 μs, then the gas has travelled 1 cm in the time it took to dissociate. If 1 cm is the distance from the shock to the vehicle surface, then the state of the gas as it hits the surface depends on how quickly it dissociates. Of course, this is a simplified example, and other processes such as recombination and stagnation would be important. Nevertheless, many conditions encountered in hypersonic flows require an understanding of the finite rate at which chemical reactions occur: the chemical kinetics of high-temperature flows.
Research at NGPDL
We are examining the vehicle geometries and freestream conditions where quantities of interest, such as heat flux, are particularly sensitive to chemical kinetics. After all, not all cases depend on the reaction rate: the density of low-altitude flight (less than about 10 km) means there are so many collisions that the gas comes to chemical equilibrium very quickly. Similarly, very high-altitude flight (above about 70 km) has so few collisions that essentially no chemical reactions occur regardless of their reaction rate. Between these extremes, chemical kinetics typically matter, but some altitudes more than others. Understanding which conditions are the most sensitive, and why, is critical for validating new chemical kinetics models.
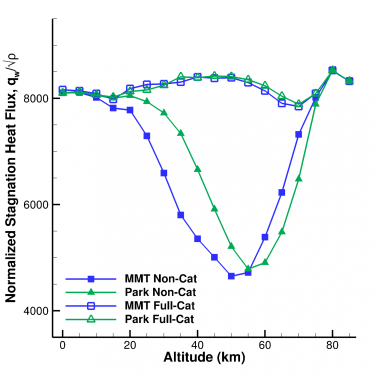
Normalized stagnation point heat flux as a function of freestream altitude, for several chemical kinetics models, from Ref. [1].
We are also collaborating with the Hanson group at Stanford, who are using shock tubes to accurately measure reaction rates. Their cutting-edge experimental results are combined with high fidelity computational models of chemical kinetics here at NGPDL.
Investigators
Acknowledgements
Funding for this work is provided by the Air Force Office of Scientific Research (AFOSR) and Lockheed Martin Corporation.
References
[1] Chaudhry, R. S., Boyd, I. D., and Candler, G. V., “Vehicle-Scale Simulations of Hypersonic Flows using the MMT Chemical Kinetics Model,” AIAA AVIATION 2020 FORUM, AIAA Paper 2020-3272, 2020. doi: 10.2514/6.2020-3272.
Introduction
Sustained hypersonic propulsion is a complex problem due to the high temperatures and velocities associated with these flows. In high-hypersonic flow regimes, combustion is limited by low residence times of the flow and the limited energy density of chemical fuel. The limits of combustion motivate using magnetohydrodynamic (MHD) body forces on an ionized gas for the conversion of power to thrust.
There have been multiple attempts at using MHD augmentation to control hypersonic flows, mostly in wind tunnel applications with various means of ionizing the working gas. An efficient way to ionize the gas for MHD acceleration is through electron beam ionization where high-energy electrons are injected directly into the flow.
Plasma Fueled Engines (PFE) offer a means of MHD acceleration for hypersonic vehicles flying at high altitudes using electron beam ionization to achieve adequate conductivity for significant MHD interaction. In PFE, the electromagnetic fields can be configured to maximize efficiency depending on the flight conditions. For lower altitudes and higher pressures, a crossed-field accelerator can be used with the electric and magnetic fields perpendicular to each other and the bulk velocity. This configuration, known as the Faraday configuration is shown below. As the vehicle climbs altitude, the electric field can tilt to match the Hall configuration seen in many popular space propulsion thrusters.
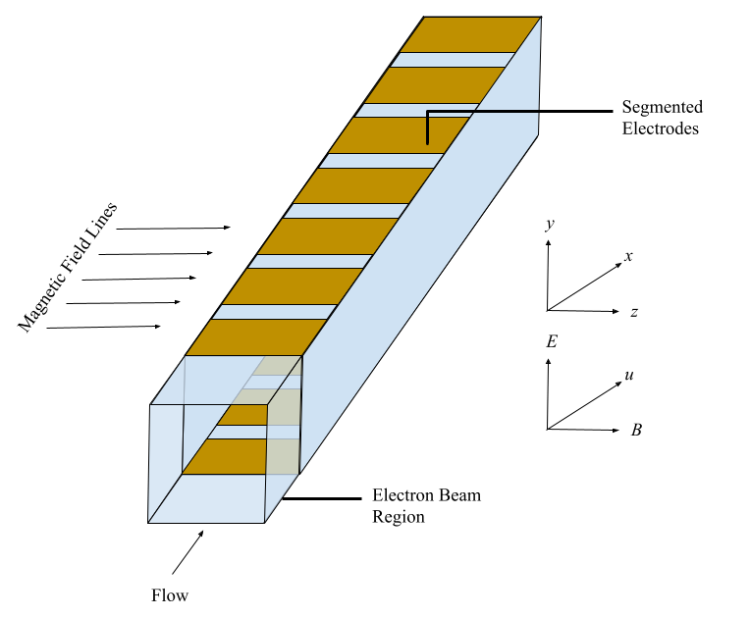
Method
In an endeavor to understand the underlying physical processes occurring in the PFE, a one-dimensional model has been developed. This model assumes that the low magnetic Reynold’s number is low so that the magnetic field, B, is prescribed throughout the entire domain. Generalized Ohm’s law can be used to calculate the current density, j, based on the electric field, E, and flow velocity, u.
The Lorentz force, jxB, and energy deposition, j∙E, are included in the Euler equations as source terms to the momentum and total energy equations, respectively.
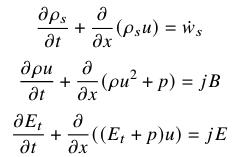
One-Dimensional Results
Solutions from the one-dimensional model show that significant acceleration is possible for a wide range of Mach numbers and altitudes. However, for large inlet Mach numbers, acceleration is preceded by an increase in temperature due to Joule heating. The figure below shows velocity, temperature, and Mach number versus velocity profiles for Mach 14 flow slowed to Mach 5.4 at the inlet of the MHD accelerator portion of the PFE. To achieve acceleration for this inlet velocity and Mach number combination, the electric field in the channel must be between 3.86 kV/m and 9.64 kV/m. Profiles with select electric field strengths in this range are shown.
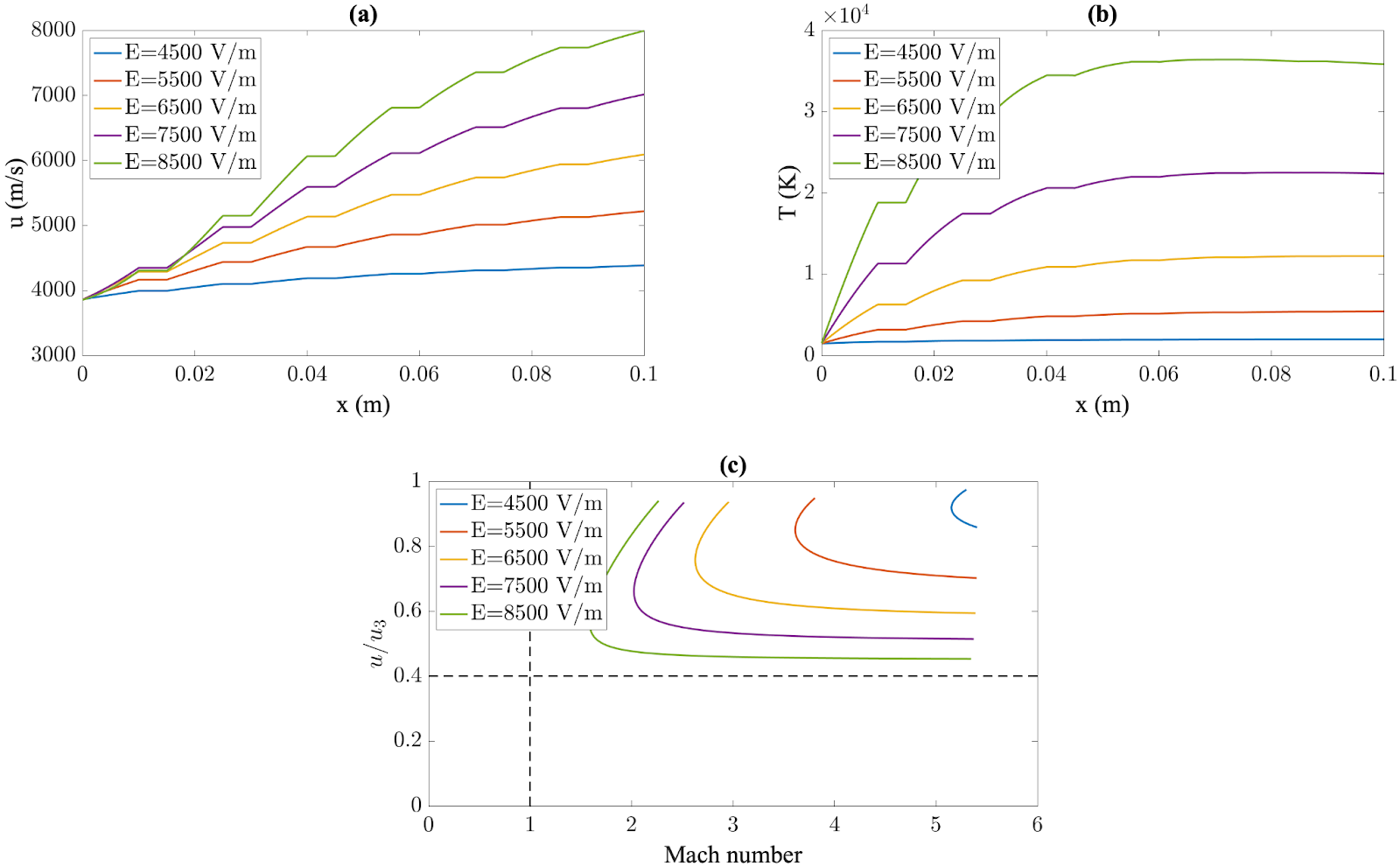
Therefore, an important task in designing PFE is tailoring the electric and magnetic field strengths to optimize acceleration down the length of the channel while keeping temperatures low.
Future work
The hypersonic CFD code developed by the members of NGPDL, LeMANS, will be used to obtain 3D solutions of the MHD accelerated flow in PFE. Furthermore, the physical models needed to understand the performance of PFE across a wide range of operating conditions will be identified using both the 1D model and LeMANS.
Acknowledgments
Financial support for this project is provided by the Lockheed Martin Corporation.
References
[1] Kava, T. E., Evans, J. A., and Boyd, I. D., "Numerical Simulation of Electron-Beam Powered Plasma Fueled Engines," AIAA 2021-3241. AIAA Propulsion and Energy 2021 Forum. August 2021.
Introduction
A promising technique for tracking boost-glide hypersonic vehicles involves detecting radio waves emitted by plasma in the wake. Passive detection would be a valuable capability, since existing tracking systems are not well suited to boost-glide vehicles. The purpose of this project is to see whether this kind of passive detection is a feasible method of tracking hypersonic vehicles, and, if it is, to characterize the signals that could be expected from different geometries and flight conditions.
There is a theoretical and experimental collaborative effort underway at CU, which seeks to provide evidence of radio emission from hypersonic objects. Modeling is handled by the NGPDL and is proceeding on two fronts: DSMC of meteoroids and CFD of hypersonic vehicles. DSMC is carried out using the MONACO software, which directly simulates trajectories, collisions, and surface interactions for rarified neutral and charged particles at high speeds. CFD is performed using LeMANS, which solves the Navier-Stokes equations for hypersonic flow in thermochemical nonequilibrium.
Entry of a meteoroid into the atmosphere is followed by ablation of the meteoroid material into the wake and subsequent ionization through high-velocity impact with the ambient atmospheric constituents. Given the rarefied nature of the ambient particles at the altitudes of interest, DSMC techniques are appropriate for simulating the interaction with the meteoroid and wake environment. Current simulations focus on meteoroids less than 1 g in mass (<8 mm diameter) entering the atmosphere at altitudes near 92 km with velocities in the range of 24-72 km/s. The simulations below show neutral (top) and plasma (bottom) densities under one set of conditions. Using an updated model of inelastic collisions, we are currently examining the sensitivity of plasma generation to factors such as meteoroid size and velocity and comparing model results with radar observations.
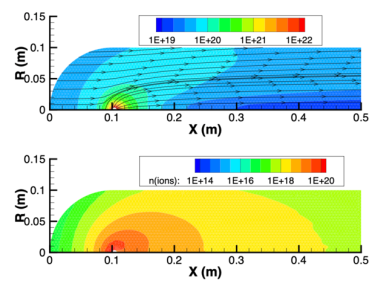
The CFD analysis focuses on full-scale hypersonic vehicles operating in the continuum regime. Preliminary investigations with SHEFEX II, a German hypersonic research vehicle, have shown that significant plasma production does not occur for extremely sharp noses (<1mm), or for flight velocities less than about Mach 10. Simulations of SHEFEX II did not show any appreciable plasma production unless the flight speed was increased far beyond reasonable expectation of a boost-glide vehicle. Below, plasma density contours are shown for a simulation of SHEFEX II and its near wake at 60 km altitude and 7.5 km/s flight speed. Even at entry speeds, the plasma production is still very low because of how slender the geometry is.
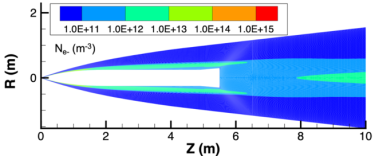
The project is now proceeding with a blunter geometry that will generate more plasma at relevant flight speeds. Once the proper geometry and flight speed have been determined, a detailed study of how different physics influence plasma formation will be performed. To start, this will include different chemistry and turbulence models. Next, the influence of ablation products in the flow will be investigated.
After refining the mechanisms of plasma generation for meteoroid and hypersonic vehicle simulations, a new electrodynamics solver will be applied to model plasma motion over irregular grids. Electromagnetic emissions from this motion can then be modeled.
Investigators
Dr. Eric K. Sutton & Timothy T. Aiken
Acknowledgements
This work is supported through funding from Northrop Grumman Corporation.
Introduction
At high enough flight velocities, the intense heating of the gas generates a plasma layer around the vehicle. The presence of free electrons in the flow field has been known to hinder the transmission and reception of radio-waves. Historically, this communications problem has been of subsidiary concern in hypersonic design as usually the more common re-entry vehicles will only undergo blackout for a relatively short timeframe, while problems such as those dealing with heat transfer take precedence. This challenge, however, is likely to be a more significant obstacle for hypersonic glide systems as a considerable amount of the mission may lie within the blackout regime, potentially complicating guidance, tracking, radar identification, electronic countermeasures, and abort functionality. Although there are currently no proven blackout amelioration techniques, many possible solutions have been proposed and tested. Of all these techniques, both passive and active, the prevailing consensus over the past fifty years has been that vehicle/trajectory selection, electromagnetic manipulation, and material injection schemes are the solutions that have shown the most promise. It is fairly evident that there will likely be no universal elegant solution to the communications blackout problem. Instead, vehicle geometry and mission requirements will likely dictate a suitable mitigation strategy. The efficacy of two technologies (electromagnetic manipulation and material injection) are computationally investigated in the context of hypersonic cruise flight following the suggested communications design engineering approach for handling blackout (AFAL, 1970), by first evaluating if communications blackout can be avoided by antenna placement or higher frequency transmissions. When this is unachievable, then two leading technologies are evaluated based on primary design drivers, specifically size, weight, energy requirements, inherent changes to infrastructure (complexity), and use of already allocated frequency spectrum.
Mitigation Techniques
Trajectory and Vehicle Selection
There are numerous uncertainties associated with modeling the aerothermochemistry of a partially ionized hypersonic flow field, including the rates at which chemical reactions take place. The rates recommended by Park have been most widely utilized. Since these rates were determined empirically from experiments ranging between 300 K and 7000 K, there are inherent uncertainties present when extrapolating to higher temperatures. A detailed assessment of Park’s chemical kinetics model for use in communications blackout simulations is performed and compared with flight diagnostics of the RAM-C II flight.
Plasma profiles perpendicular to the RAM-C II wall are constructed and the maximum electron density is found from these lines for each axial reflectometer station location. A direct comparison between the maximum electron number density generated through trajectory point simulations and the reflectometer data is made to eliminate the assumptions inherent with interpolation. The reflectometer measurements have with horizontal bars depicting the altitude range in which the reflection coefficient experienced a definite rise. Comparisons are made between models using different number of species and different sets of forward reaction rate coefficients for the second reflectometer station, shown in the figure below. Detailed comparisons with all four reflectometers can be found in Ref. [1].
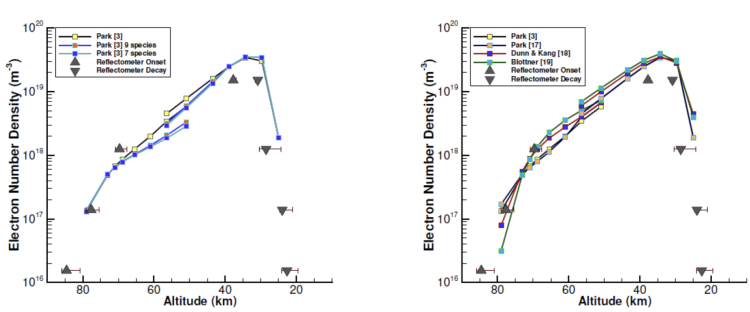
Direct comparisons of the maximum electron number densities calculated with the various equilibrium constants are made with the available electrostatic probe rake data (located 1.234 m downstream of the stagnation point). Both probes 1 and 8 are used here for comparison. The differences brought upon by the variation of the equilibrium constant models are displayed in the figure below and identify quantify the altitudes where recombination of electrons dictates the plasma flowfield. Whereas the electron densities calculated using Kim’s and Gordon & McBride’s equilibrium constants stay within 7% of each other throughout the trajectory, there is considerably more disparity between these models and that of Park’s, especially at low altitudes. In these low altitude regimes, the maximum temperatures near the electrostatic probe can be as low as 3,000 K. The differences of the equilibrium constants at low temperatures can play a significant role in electron concentrations.
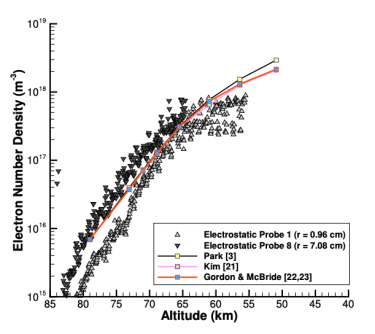
Comparisons between the inferred electron number density readings of stations 1 and 8 of the electrostatic probe rake and the predictions obtained with different equilibrium constant models [1].
Electromagnetic Manipulation
The exploitation of electromagnetic fields as a blackout amelioration technique dates back to the early 1960s, when Hodara proposed that static magnetic fields could be used to create a window through which RF transmission can pass through will limited attenuation. By orienting the magnetic field lines such that the motion of the electrons is constrained through gyration, the dispersion relation becomes altered where a wave below the plasma frequency can propagate. The magnetic field limits the transverse motion of the electrons, and for an infinitely strong magnetic field, no interaction would take place between the traverse wave and electrons. This technique is one of the few to have been tested in flight, as signal attenuation and antenna impedance measurements were made during the RAM A-2 flight to determine the effectiveness of a magnetic window. Permanent magnets within the constraints of a hypersonic vehicle are limited to around 0.15 T and are hampered by the Curie temperature. With current technologies, a superconducting electromagnet would weigh over 500 kg and be difficult to implement. Nevertheless, many methods to reduce the magnetic field strength requirements and subsequently the system weight requirements have been proposed. For instance, the hybrid use of electrostatic fields has also shown promise, incorporating both magnetic window and electrostatic collection schemes. This system utilizes crossed electric and magnetic fields to collect both electrons and ions and accelerate the charged particles past the antenna, lowering the adjacent electron number density. This ExB drift technique has been demonstrated by Kim for use in blackout mitigation as seen in the figure below [2].
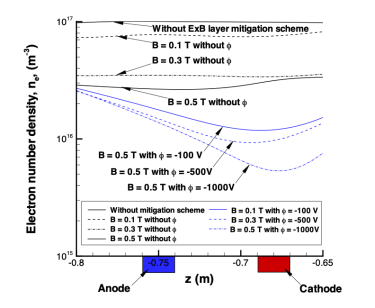
The electron number density distribution near the surface of OREX for several operational conditions of an E x B mitigation scheme[2].
Material Injection
Injection of material to the plasma flow upstream from the antenna has been consistently listed as one of the most promising techniques for alleviating the effect of the plasma layer. Among other factors, favorability to this mitigation technique can be largely attributed to its extensive testing in a number of flights, with several liquid injection experiments having been conducted during the RAM, Trailblazer, and Gemini flights, demonstrating varying levels of success. Injection of thermal quenchants lowers the temperature of the flow which in turn lowers the electron number density required to maintain equilibrium. As dissociating reactions are generally endothermic, chemicals with high heats of atomization are used as quenchants, such as water, propane, and freon variants.
As the water injection technique is one of the few with published flight data, it is beneficial to first validate the current numerical methodology. As such, comparisons with available flight data from the RAM-C I flight, specifically that of the electrostatic probe, are made, as shown in the figure below.
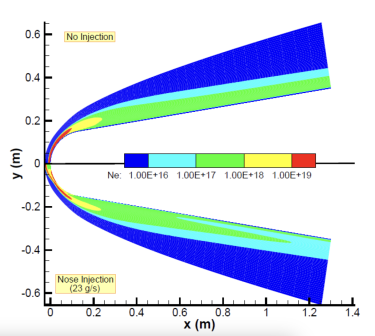
Electron number density of RAM-C I at 61km with no injection (top) and injection at the stagnation point; x = 0 cm (bottom).
Investigators
Pawel Sawicki
Acknowledgements
Funding for this work is provided by L3Harris Technologies.
References
[1] Sawicki, P., Chaudhry, R. S., and Boyd, I. D., "Influence of Chemical Kinetic Models on Plasma Generation in Hypersonic Flight", AIAA Journal, 2021
[2] Kim, M. K., "Electromagnetic Manipulation of Plasma Layer for Re-Entry Blackout Mitigation", Ph.D. thesis, Department of Aerospace Engineering, University of Michigan, 2009.