Kinetic Simulations of Thermionic Hollow Cathode Plasmas
Background:
Ubiquitous in the field of electric propulsion, thermionic hollow cathodes are electron sources which utilize thermionic emission from a low work function insert material to achieve large extracted currents. A neutral propellant, often xenon, is flowed through the cylindrical device and ionized by the electron current from the walls, thus creating a quasi-neutral plasma which is extracted through an orificed endplate. Hollow cathodes are used as part of both Hall effect and gridded ion thrusters, which are some of the most common forms of electric propulsion in use today.
The operation of hollow cathodes, despite their prevalence on operational spacecraft, is still not entirely understood. For example, erosion of the cathode keeper face in long-duration wear tests causes premature device failure, indicating that high-energy ions impinge on its surface. Recent experimental data provides strong evidence for the existence of a plasma instability, the ion acoustic instability, in the cathode plume region. As this instability is inherently kinetic in nature, it cannot be modeled from first principles using existing fluid simulation codes. Therefore, closure models are currently used in these codes which approximate the bulk effects that this instability has on the cathode plasma. These closures have significantly aided agreement of simulations with experimental data, further reinforcing the theory of this instability's existence in the cathode plume region.
Despite recent advances in understanding the physical processes which occur in hollow cathodes from both theoretical and reduced-order modeling approaches, there currently exist very few endeavors to understand the cathode plasma through high-fidelity, kinetic simulations. This avenue of research is important to providing a complete picture of the hollow cathode plasma, as it allows insight into the state of electron and ion velocity distribution functions (VDFs) which govern bulk (fluid) properties. Should these distributions by far from their equilibrium state, for example, this would allow resolution of small populations of energetic ions which may be responsible for large measured erosion rates. Therefore, this research aims to derive a more complete understanding of the non-equilibrium hollow cathode plume plasma through kinetic simulations.
Methods:
This research utilizes a deterministic approach through directly resolving the Vlasov, or collisionless Boltzmann, equation with a finite volume method. The method is second-order accurate in space and uses the explicit Runge-Kutta (RK) method in time. To resolve the electric field, Poisson's or Ampere's equations are solved concurrently. Collisions may be included via the Bhatnagar-Gross-Krook (BGK) operator. Both hybrid-kinetic and fully-kinetic methods are developed in this work; the hybrid-kinetic method utilizes a Boltzmann relation model for electrons while solving the Vlasov equation for ions and neutrals.
Hybrid-Kinetic Results:
An axisymmetric implementation of the Vlasov equation is implemented, with unique schemes in place for both ions and neutrals. The solvers are benchmarked with the direct simulation Monte Carlo (DSMC) code, MONACO, for the NSTAR discharge cathode geometry.
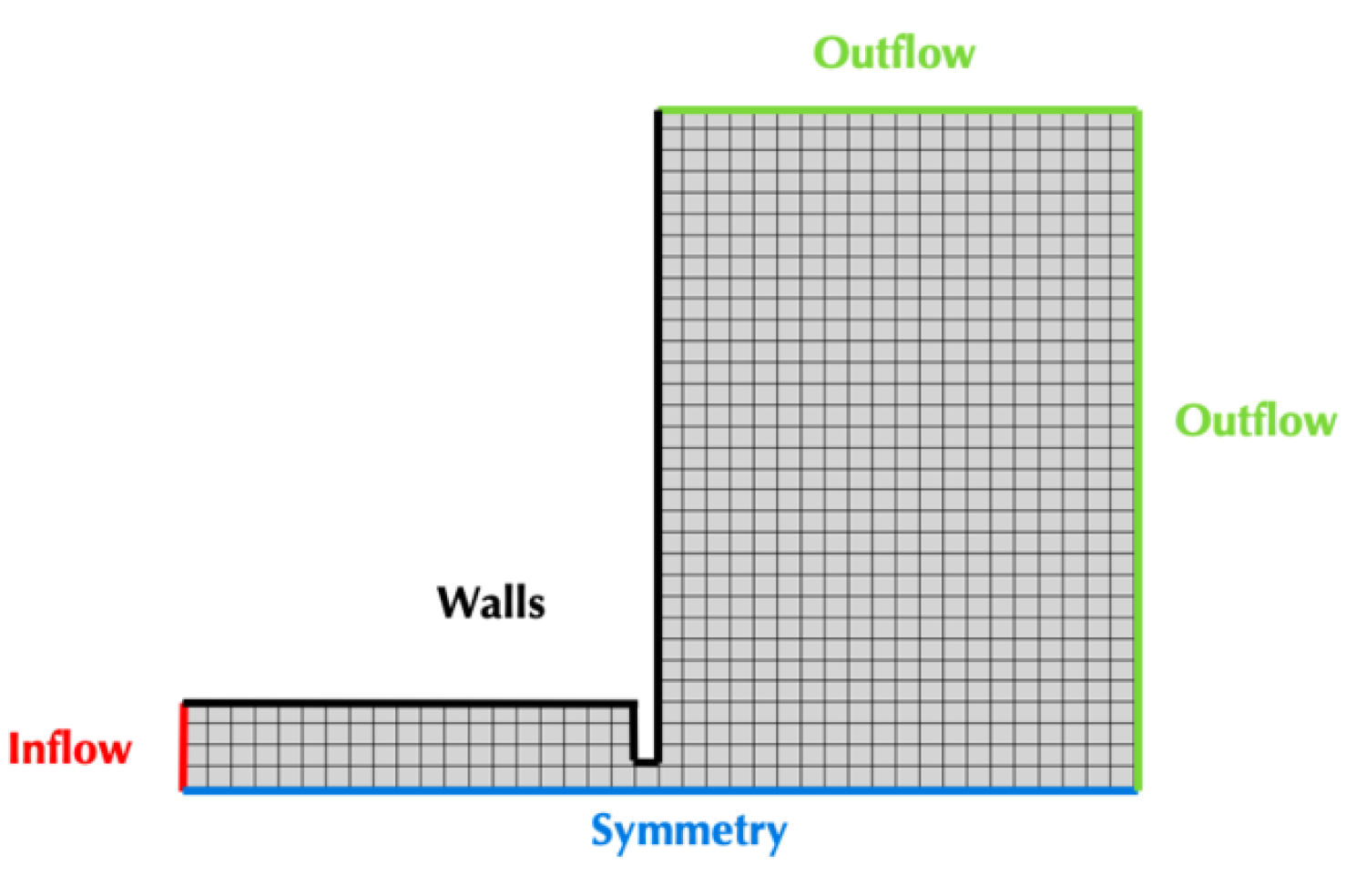
Figure 1.
Results demonstrate good agreement for a collisional, axisymmetric neutral flow. Results are then compared with experimental data and an existing fluid code for a coupled neutral+plasma flow. Decent agreement is found near the orifice and into the plume, while for the emitter (interior) region additional physics needs to be included for better agreement. Figure 2 gives comparison for the neutral flow benchmark, while Figure 3 compares plasma densities between experiment, fluid code, and hybrid-kinetic code for the NSTAR cathode operating at the TH15 condition.
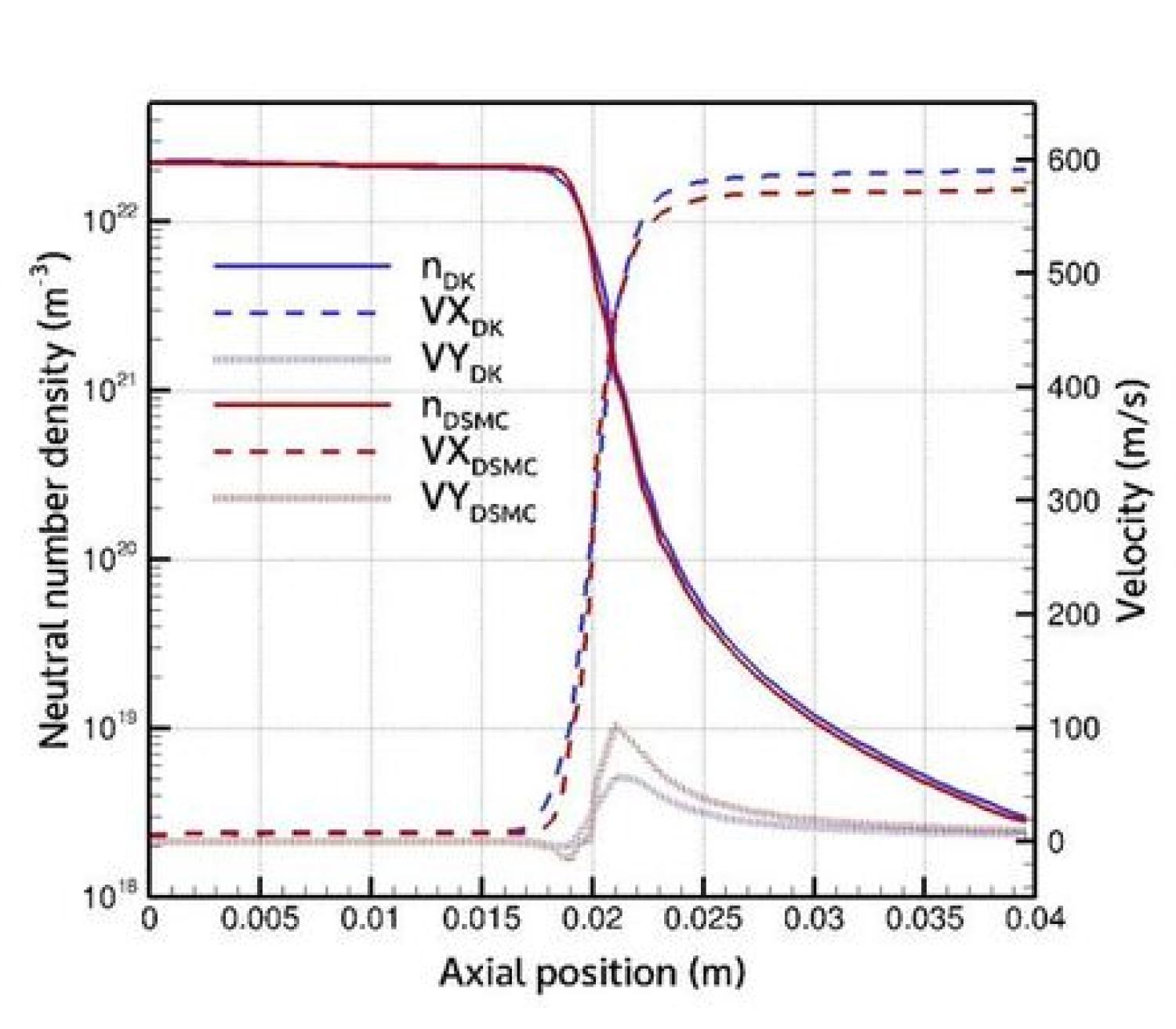
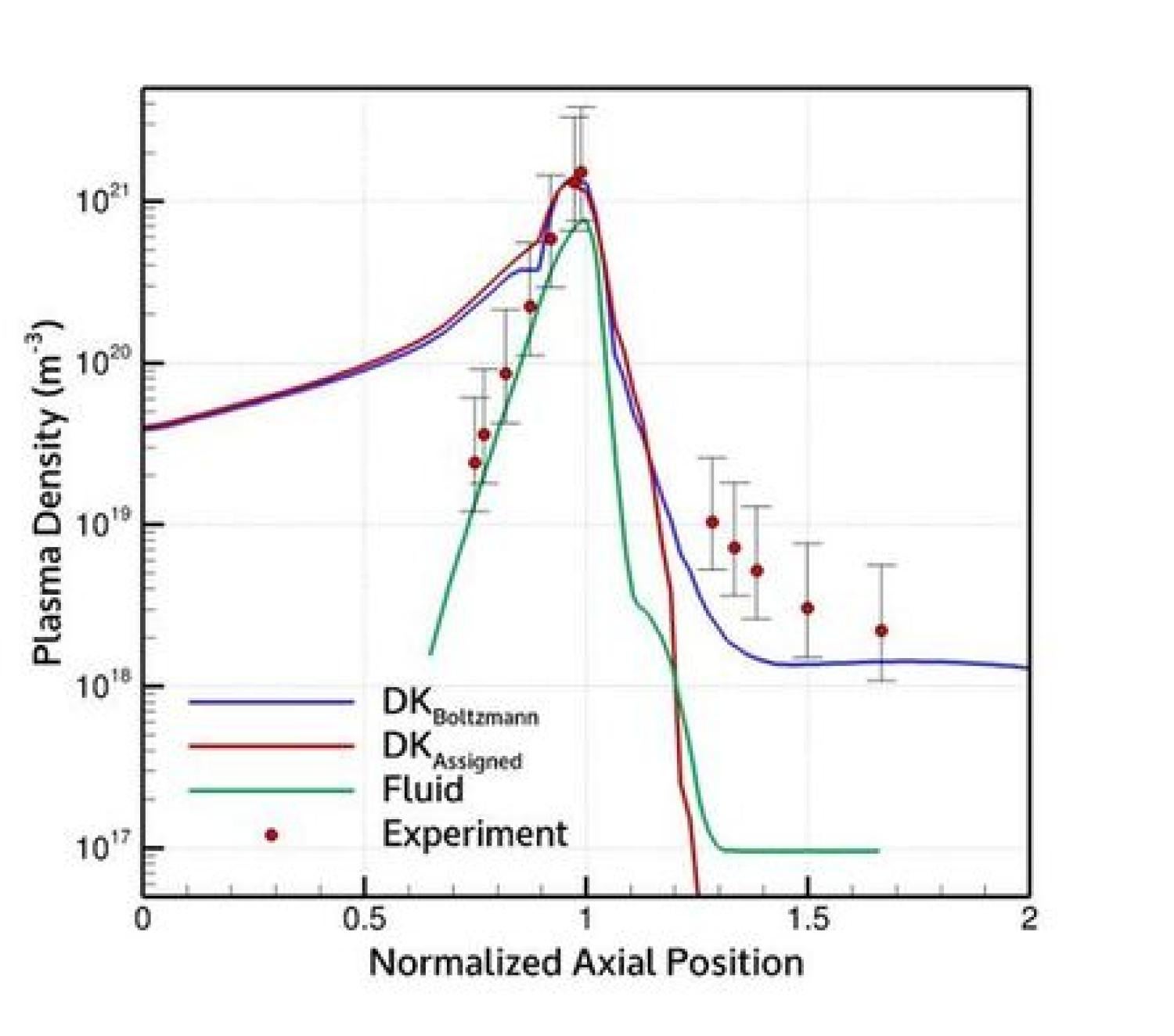
Figure 2. Figure 3.
Full-Kinetic Results:
Fully kinetic, 1D1V (one spatial dimension, one velocity dimension) Vlasov-Poisson simulations are conducted for a current-carrying plasma such as that found in the hollow cathode plume to analyze the effect of the ion acoustic instability. Using periodic boundary conditions, a test case is set up using an externally applied electric field to emulate the potential gradient in the cathode plume. In addition, the effects of momentum exchange collisions are included; with both of these effects included, the electron drift may reach a steady state, provided no instability should occur. Should the ion acoustic instability grow, a quasi-steady state is still found for the electron drift.
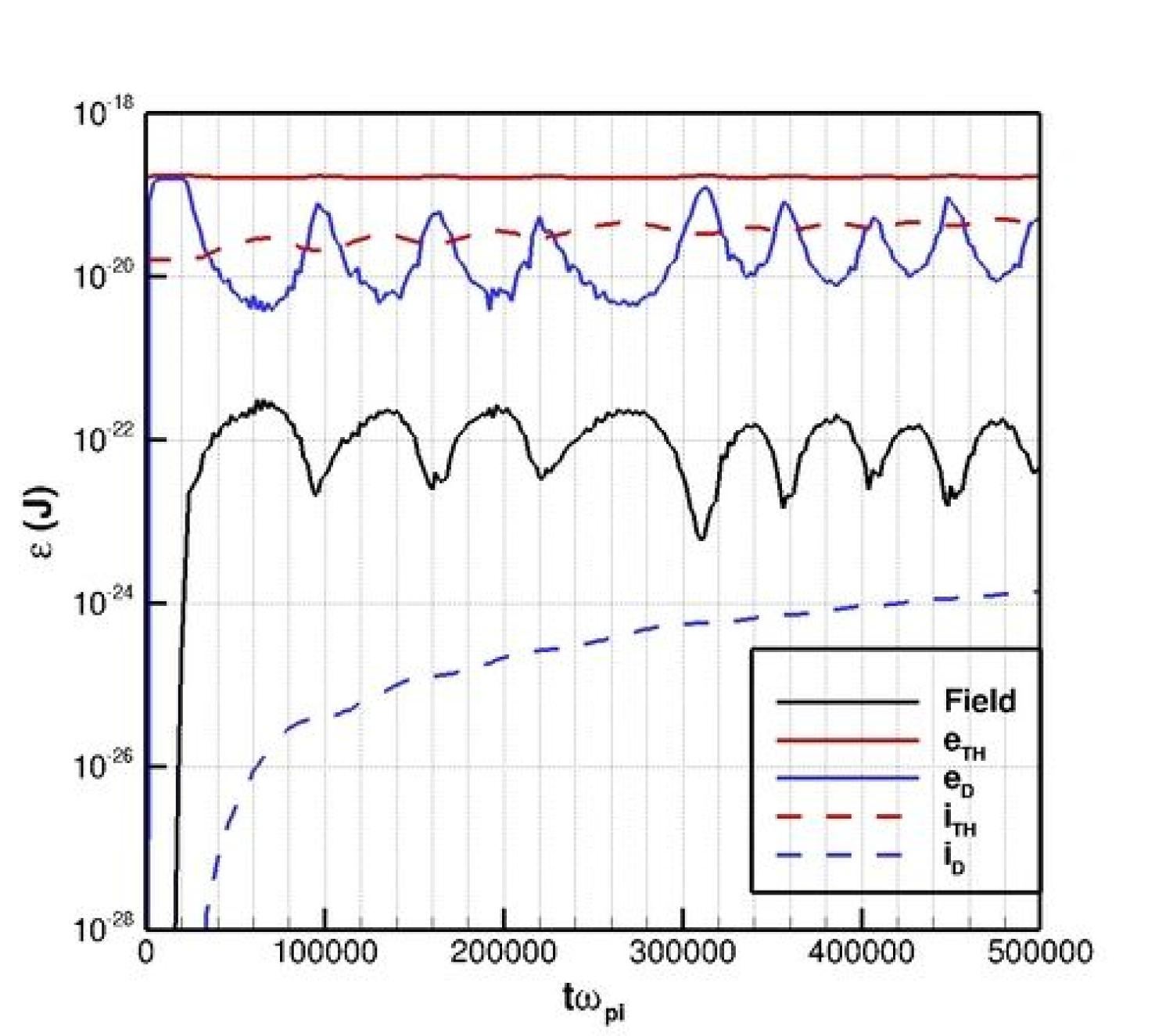
Figure 4.
Figure 4 demonstrates the distribution of system energies over the course of the simulation; on average, electrons maintain their drift and thermal energies, while ions demonstrate an increase in both types of energy. Electrons reaching a steady-state drift allows for an effective anomalous collision rate parameter to be calculated due to the ion acoustic instability. This parameter is compared with two fluid closure models used in the literature; one of these closures, the Sagdeev-Galeev (SG) model is the simpler of the two, being based primarily upon the electron drift velocity, U_e. The Davidson-Krall (DK) model, on the other hand, uses the electrostatic field energy to estimate the collision rate, and is found to agree quite well with the numerically estimated values.
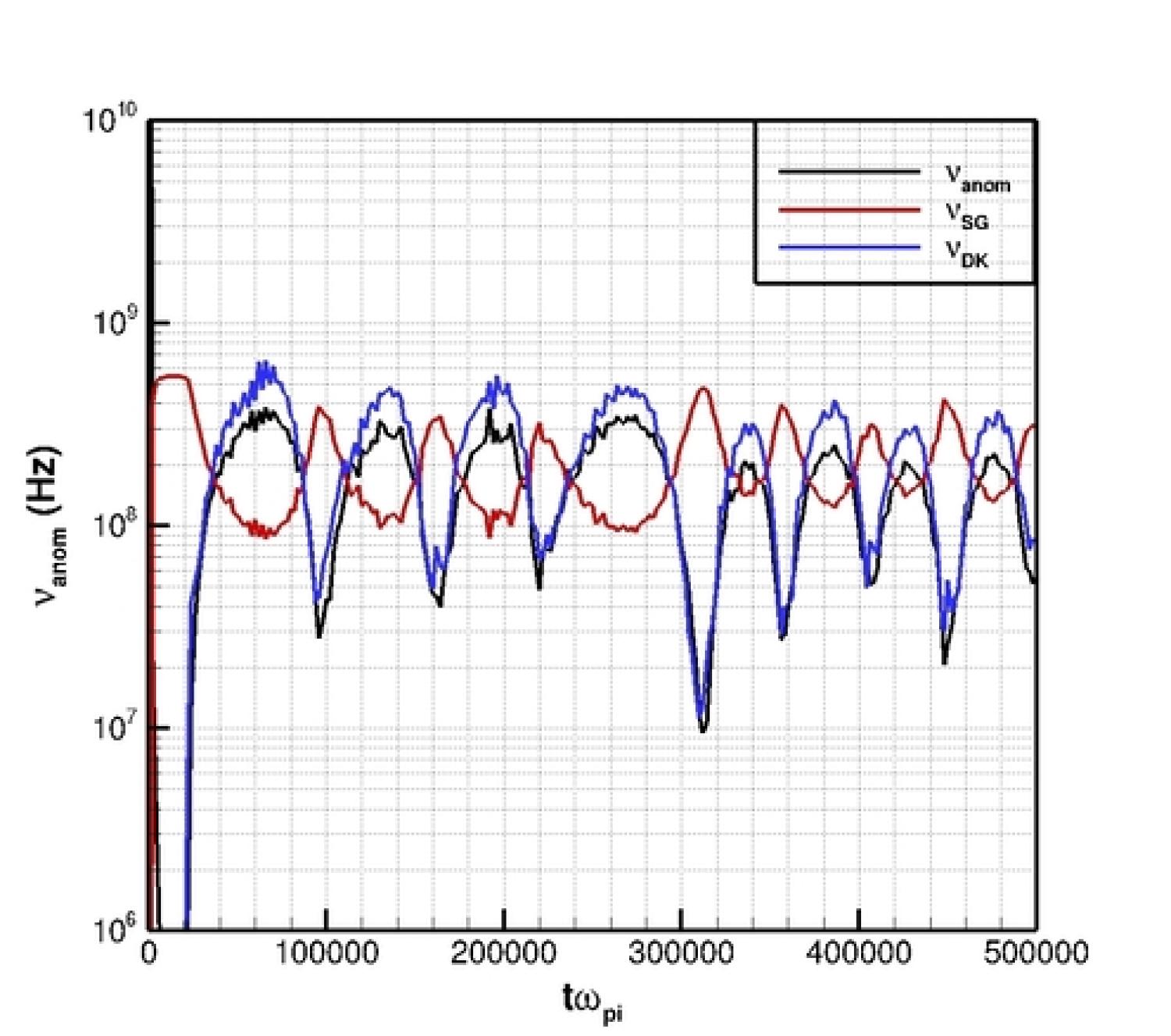
Figure 5.
Figure 5 demonstrates the close agreement of the DK model with numerically estimated collision rate values, while highlighting the shortcomings of the SG model.
Future Work:
Current fully kinetic simulations have utilized periodic boundary conditions, which prevents a better understanding of long-time duration behavior. In the future, simulations will utilize open boundary conditions, in which the plasma enters and exits the domain. In addition, the affect of double layer formation and multiply charged ions on the hollow cathode plasma are subjects of current study.
Investigators:
Alexander R. Vazsonyi
Acknowledgements:
This work is supported through the National Defense Science and Engineering Graduate (NDSEG) Fellowship sponsored by the Department of Defense.