Research
This page tries to reflect some of my research topics in simple language. My research is mainly focused on risk, resilience, and safety assessment of infrastructures (e.g., concrete dams, nuclear power plants, bridges, and towers) under extreme environmental conditions (e.g., earthquake, flood) and evolving situations (e.g., material deterioration and climate change).
Dams and Levees
Safe operation, proper maintenance, and preventing potential dam failure are critical tasks for dam owners and society. Dams are typically categorized into four groups based on their hazard potential: 1) high, 2) significant, 3) low, and 4) undetermined. Based on the latest report by the US Army Corps of Engineers (USACE), the number of dams in each one of those four categories approximately is 14700, 12400, 59000, and 1300, respectively. Figure 1 shows the distribution of hazardous dams in the US. The number of high-hazard dams is increased by about 40% in the past decade. Over 70% of the total dams in the US are 50+ years old. The Association of State Dam Safety Officials (ASDSO) estimates that it will require an investment of $21 billion to repair these aging, yet critical, high-hazard dams.
Figure 1: Distribution of hazardous dams in the US [FEMA-956, 2013]
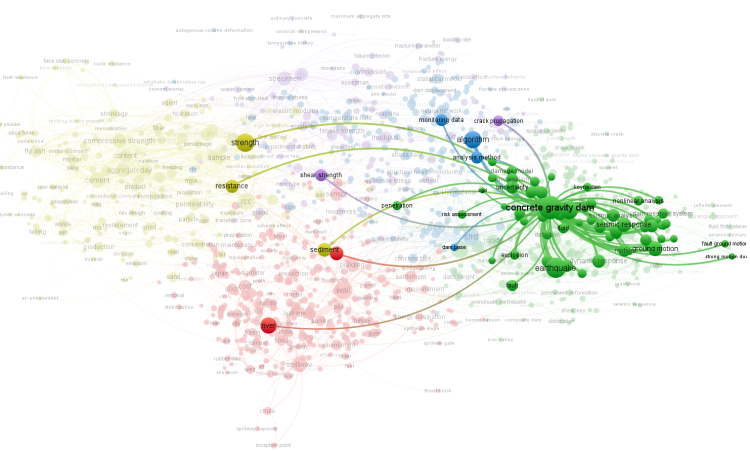
Figure 2: Visualizing the bibliographic database related to concrete dams
Potential Failure Modes (PFM)
A dam PFM is a chain of events leading to unsatisfactory performance of the dam which could lead to the uncontrolled release of the reservoir water (FERC, 2005). Failures of dams start with some initiating event that causes an adverse change in the structure, e.g. seismic, flooding, human interaction, or landslides. The figure below illustrates the major PFMs for three types of concrete dams (i.e. gravity, arch, and buttress dams). PFMs are mainly overstressing, sliding, joint opening, tensile cracking, buckling, concrete crushing, racking, and localized or global failures.
Figure 3: Potential seismic failure modes of concrete dams [Hariri-Ardebili, 2015; PhD Dissertation]
Detailed Deterministic Analysis
The figure below illustrates a methodology for comprehensive seismic safety evaluation of existing concrete dams. First, the finite element model is defined and all the nonlinearity sources (material, joints, geometry), as well as interactions (fluid-structure and soil-structure), are applied in the model. Coupled or uncoupled thermo-mechanical analysis is implemented to realize the performance of the dam at the pre-earthquake condition. Pre-earthquake safety assessment of the concrete dams may be conducted for a relatively long period of time (based on the seismicity condition of the site). In such a case, safety evaluation analysis should include the aging constitutive models (e.g. creep and alkali-aggregate reaction). Second, seismic analysis is performed considering the calibrated parameters obtained from the previous step. An appropriate main shock is selected based on the event that has the most contribution to ground-motion exceedance hazard in terms of moment magnitude and source-to-site distance. The selected ground motion can then be scaled using the uniform hazard response spectra. Third, post-seismic analysis is performed taking into account the condition of the dam at the end of the main shock. Static stability analysis and seismic analysis using the aftershock event(s) are required to insure the safety of the dam.
Figure 4: A comprehensive methodology for deterministic safety evaluation of the existing concrete dams [Ref-J-037]
Performance-based and Probabilistic Assessment
Although a comprehensive deterministic seismic analysis provides useful information about the performance of the dam, the contribution of uncertainty sources makes the problem more complex and challenging. In general, random variables (RVs) can be categorized as aleatory (intrinsic randomness of a phenomenon) or epistemic (lack of knowledge). Thus, the performance of the dam is evaluated more precisely in the context of probabilistic approaches (e.g. performance-based earthquake engineering – PBEE). The Pacific Earthquake Engineering Research (PEER) PBEE framework, Figure below, facilitates direct calculation of the effects of uncertainty and randomness on each step in the performance-based procedure.
Figure 5: General framework of PEER PBEE methodology [Porter, 2003; ICASP9]
A comprehensive probabilistic safety evaluation of dams should account for the material/modeling uncertainty as well as external loading. This should be combined with an appropriate structural analysis method (depending on the complexity of the model and the required accuracy) as shown in Figure below.
Figure 6: Progressive analysis methodology for seismic assessment of structures [Ref-J-030]
The outcome of nonlinear structural analysis is a capacity curve or capacity surface which shows the progressive failure of the structure under different earthquake intensities. An advanced structure-dependent hybrid damage index is required to track the evolution of damage in concrete dams. Some samples are shown in the figure below.
Figure 7: Concept of damage index and progressive failure in concrete dams [Left: Ref-J-038; Right: Ref-J-046]
Incorporating the uncertainties in nonlinear structural analyses leads to the derivation of fragility curves or surfaces. A fragility function represents the probability of exceedance of a specific limit state (or collapse) conditioned on some environmental actions (e.g. earthquake). The figure below illustrates the derivation of fragility functions for a gravity dam considering the uncertainty in the dam-foundation interface joint (RVs are correlated and sampled based on LHS) and ground motions (a large set of signals are selected based on PSHA).
Figure 8: Fragility curve and surface for concrete dams accounting for both the uncertainty types [Ref-J-047 & Ref-J-049]
Nuclear Power Plants
Nuclear power in the US provided about 20% of the total electric energy in 2014. According to the Atomic Energy Act of 1954, and NRC regulations, the operating licenses for commercial power reactors are issued for 40 years and can be renewed for an additional 20 years, with no limit to the number of renewals. The original 40-year license term was selected on the basis of economic and antitrust considerations rather than technical limitations. Henceforth, many plants have sought (and obtained) a 20-year life extension. In the US, the average structural life is 32 years. Also in the US, most NPPs have already had a life extension from 40 to 60 years, and serious consideration is now being given to a further extension to 80 years. Thus, a comprehensive evaluation of potential aging-related degradation modes for light-water reactor materials and components is required [Ref-J-036].
ASR Affected Concrete Components
Alkali-Silica Reaction (ASR) is a deleterious reaction in concrete that causes long-term swelling of the concrete and is very likely to affect the structural integrity of the structure (serviceability/cracking and/or strength). Though not yet well-publicized (for obvious reasons), some nuclear reactors are starting to show signs of ASR. The aim of this research is to step by step analysis of several reinforcement concrete components as part of a nuclear power plant suffering from ASR as shown in Figure below. Different models and assumptions are considered for finite element modeling and capacity curves are derived under monotonic and cyclic loading.
Figure 9: Shear capacity of ASR affected reinforced concrete components [Saouma et al. 2015; ORNL]
Seismic Assessment of ASR-Affected Nuclear Containment
The objectives of this research are to 1) perform modern nonlinear transient 3D finite element analysis of an NPP with deconvolution, free field, soil-structure interaction, and fracture mechanics-based model to account for both cracking and uplift; 2) explore the applicability of PBEE paradigm for the safety assessment of NPP; 3) quantification of all uncertainty sources and deriving the fragility function; and 4) accounting for the age-dependent material deterioration in capacity functions.
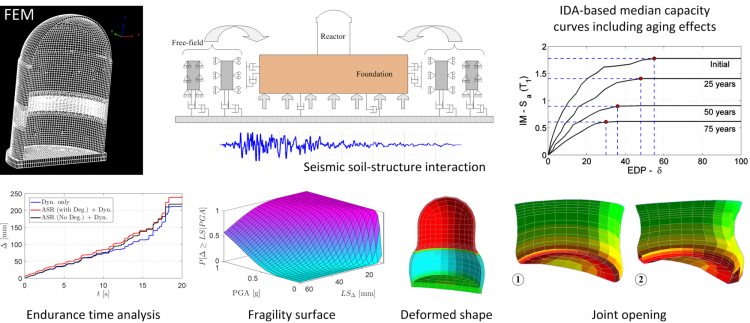
Figure 10: Comprehensive seismic safety evaluation of nuclear containments with aging effects [Saouma and Hariri-Ardebili, 2016; NRC]
Kinetics of ASR Reaction and Life Prediction
ASR expansion is a function of many parameters among them time, temperature and relative humidity (RH) play key roles, Figure 10 (left plot). Once ASR is known to occur, two questions take on paramount importance: 1) How will ASR affect structural integrity and safety? and 2) For how long will the reaction continue? This research tries to answer the second question using an innovative technique that combines field observations, laboratory tests, and optimization algorithms. A sample prediction based on an unconstrained optimization technique is shown in the Figure below (middle plot).
Starting from the chain of three chemical reactions which characterize the ASR, this research attempts to develop a mathematical framework through which the reaction kinetics can be better understood. First, an analytical solution is sought. Though one was not found, three new conservation laws were derived. Then a numerical solution is applied, and important observations are made, Figure 10 (right plot).
Figure 11: Prediction of residual life in ASR-affected structures (left); Kinetics of ASR reaction (right) [Ref-J-043]
Towers
The aim of this research is to evaluate the fragility and vulnerability of infrastructures against earthquake ground motions. An innovative structural analysis technique is used (i.e., Endurance Time Analysis) to facilitate the nonlinear transient analysis and to reduce computational costs.
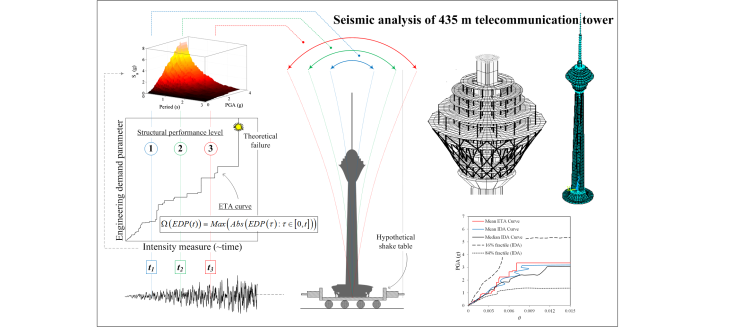
Figure 12: Safety evaluation of a telecommunication tower against the earthquake hazard [Ref-J-033]
Bridges
The aim of this research is to quantify the AAR damage in a viaduct bridge through a comprehensive uncertainty quantification of the random variables. An efficient sampling technique is used to account for the material degradation and concrete nonlinear damage properties. Non-uniformity in the AAR expansion at different sections of the viaduct is a key parameter in the classification and crack propagation.
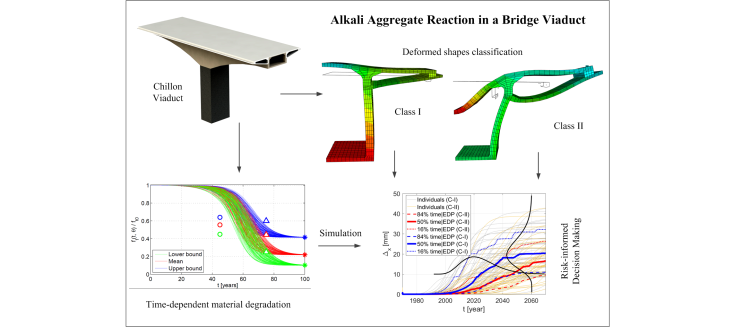
Figure 13: Uncertainty quantification of AAR expansion in a bridge viaduct [Ref-J-058]
Surrogate Models in Reliability Analysis
A surrogate model is an engineering technique that is used when the outputs of a process cannot be directly measured or related to the input parameters. Different techniques do exist for data classification or prediction of the future trend.
Machine Learning for Multi-Hazard Analysis of Dams
Machine learning techniques are basically used either for regression or classification purposes. The aim of this research is to investigate the application of different machine learning techniques (e.g. support vector machine, naive Bayes classifier, and K-nearest neighbor) in the reliability analysis of concrete dams. The failure probability is computed for multi-hazard scenarios (including hydrological, seismic, and aging). A hybrid method of finite element and machine learning is then proposed for dam safety evaluation.
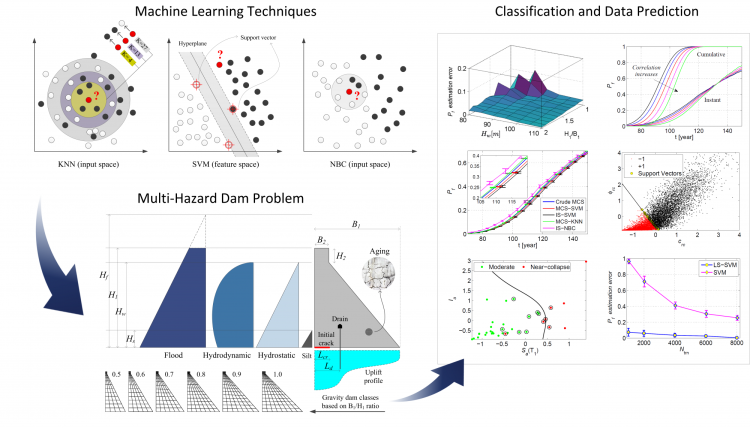
Figure 14: Reliability analysis of dams with machine learning techniques [Ref-J-055, Ref-J-056]
Response Surface and Optimal Design of Experiments for Dams
A metamodel (or model of the model) is a mathematical approximation of the implicit simulation code. Outputs are presented in a closed-form function of input parameters. Response surface is a technique to develop analytical meta-models. By definition, the design of experiments (DOE) refers to a statistical technique that systematically defines the minimum (or efficient) number of experimental sampling points to optimize the computed or observed responses. These techniques are applied to both gravity and arch dams. Linear elastic and nonlinear damage analyses are performed. The accuracy of the proposed models is compared to the crude Monte Carlo simulation or Latin Hypercube sampling. Finally, the reliability of the system is evaluated.
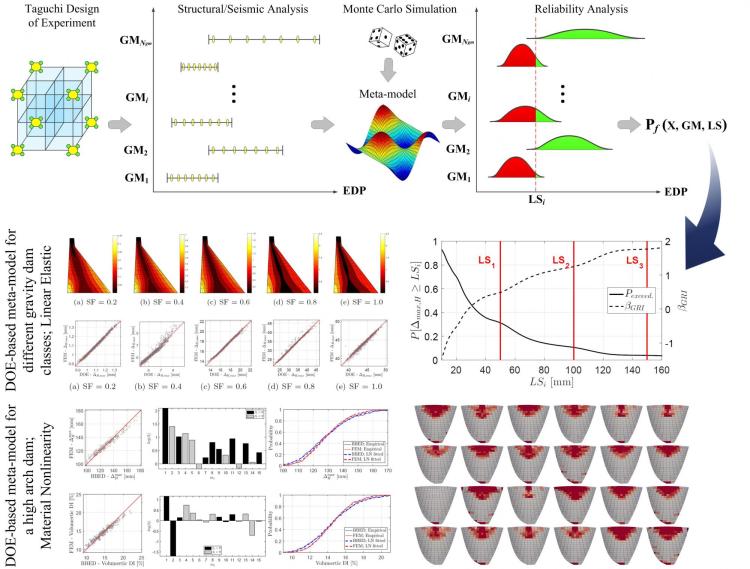
Figure 15: Response Surface metamodel for concrete gravity and arch dams [Ref-J-059, Ref-J-060, Ref-J-063]
Force Density Ratios of Fabric Membranes
While architectural fabric membranes are used as efficient cladding to cover large areas, they also have structural performance. Since there is a direct relationship between "form" and "force" distribution in tension membrane structures, the form-finding procedure is an important issue. Ideally, once the optimal form is found, a uniform pre-stressing is applied to the fabric which takes the form of a minimal surface. This paper evaluates the optimum amount of force density ratio considering the curvature of the flexible borders for structural configurations, i.e. hyper and conic membranes.
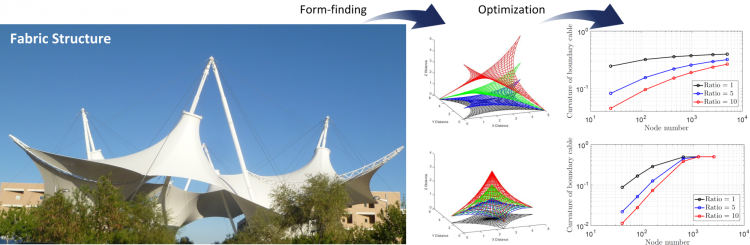
Figure 16: Optimal force density ratios of flexible borders in fabric structures [Ref-J-065]
Experimental Tests and Monitoring
The objective of these tests is to understand the structural response of full-scale and scaled-down structural components through a series of laboratory experiments.
Steel Plate Shear Wall
The applicability of an enhanced modular steel plate shear wall is tested under cyclic displacement. Different failure modes were identified, and the load-displacement capacity curves were obtained. The proposed modular wall can be replaced quickly in any framed structure and improves the lateral resistance of the building.
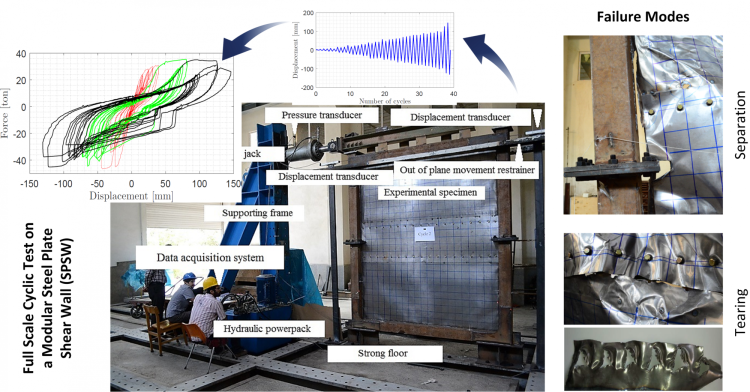
Figure 17: Full-scale experimental test on steel plate shear walls [Ref-J-xxx]
Shear Test on ASR-Affected Concrete Blocks
This NRC-funded project [led by Prof. Saouma] aimed to develop a concrete mix design that will expand by approximately 0.5% in 6 months and has a compressive strength of around 4,500 psi, a 3-inch slump, and low air content. Cast many cubes (with various uniaxial, biaxial, or triaxial reinforcements), and shear specimens to monitor the ASR expansion, and cure them under controlled conditions. Assess both expansion and crack indices. Assess shear strength degradation of plain and structural concrete subjected to ASR. Validate the Experimental tests with numerical simulations (Figure 16).
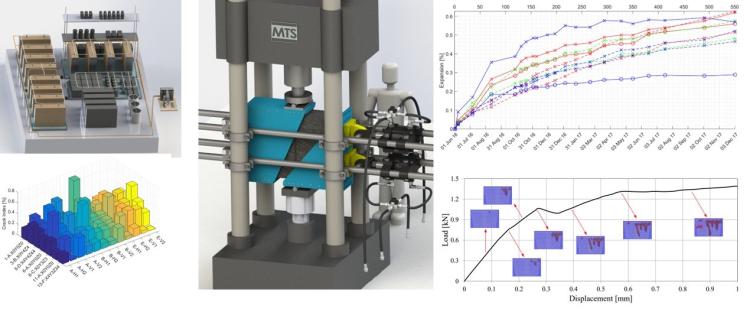
Figure 18: Experimental test on the shear capacity of ASR-affected concrete specimens including the numerical simulation [Ref-J-xxx]
Instrumented Health Monitoring of an Earth Dam
Structural health monitoring is a crucial step in the safe operation of infrastructures. The long-term stability of an earth dam was investigated using multiple instrumentations. Various quantities were measured such as pore water pressure, water level, and internal stress ratios using inspection devices such as ordinary and Casagrande piezometers, and total pressure cells. Overall, the condition of the case study dam was assessed to be normal.
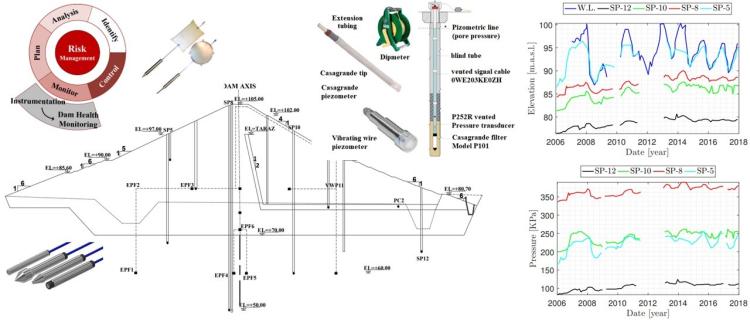
Figure 19: Instrumentation map including the devices and the measurements [Ref-J-xxx]
Material Heterogeneity and Random Fields
Many of the concrete structures are aging and their material properties are different from the design ones. Moreover, the aging process of heterogeneous in time and space, and thus, it creates non-homogeneous patterns in different length scales. The objective of this research is to understand the macro-scale structural responses of mass concrete structures with heterogeneous material properties. Studies are so far classified into four groups: 1) vibration characteristics of the (six) high arch dams and proposing a new relationship for the vibration period as a function of dam class, material properties, and randomness; 2) Linear elastic analysis of 2D gravity dams with concrete random fields; 3) nonlinear damage analysis of 3D slice gravity dams with heterogeneous concrete subjected to the intensifying acceleration function; 4) ASR analysis of an arch dam with heterogeneous expansion and realistic transient thermal analysis.
Overall, all this research confirms that macro-scale material heterogeneity and random fields affect the structural responses and may change the potential failure modes.
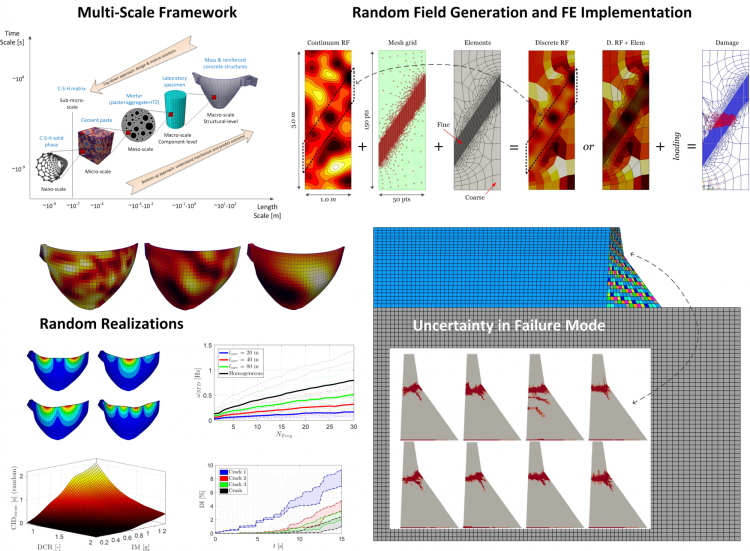
Figure 20: Multi-scale generation of random fields for structural analysis of concrete dams and concrete components [Ref-J-xxx]