Research Projects
Here are some brief summaries of some of the current and recent research projects to give you at least a flavor of the type of research we are doing in the lab.
Artifical Gravity as a Countermeasure to Spaceflight Physiological Deconditioning
During space exploration missions, astronauts experience physiological deconditioning across a wide range of systems, including bone loss, muscle weakening, cardiovascular deconditioning, fluid shifts, visual impairment, and sensorimotor/neurovestibular alterations. While a variety of countermeasures (e.g. aerobic exercise, fluid loading, lower body negative pressure) have been proposed to address individual physiological systems, they tend to be, at least partially, ineffective. Artificial gravity (AG), produced through centrifugation, has been proposed as a universal countermeasure to prevent physiological deconditiong across multiple physiological systems (see Artifical Gravity by Clement and Bukley). However, a centrifuge has never been tested on humans in spaceflight as a countermeasure and there remain numerous design considerations. For example, what gravity level is necessary to prevent deconditioning: 1 Earth G or would a partially gravity level be sufficient? Must the centrifugation be continuous or could it be intermittent? How small of a radius is tolerable before the high spin rate causes Coriolis forces to affect arm and leg movements and vestibular cross-coupling to provoke disorientation and motion sickness? We aim to investigate these and related questions using ground-based centrifuges. Current experiments on the Human Rotator Device (GyroStim/UltraThera Technologies, Inc.) in our lab are investigating the tolerable rotation rates prior to which the vestibular cross-coupling illusion becomes noticeable.
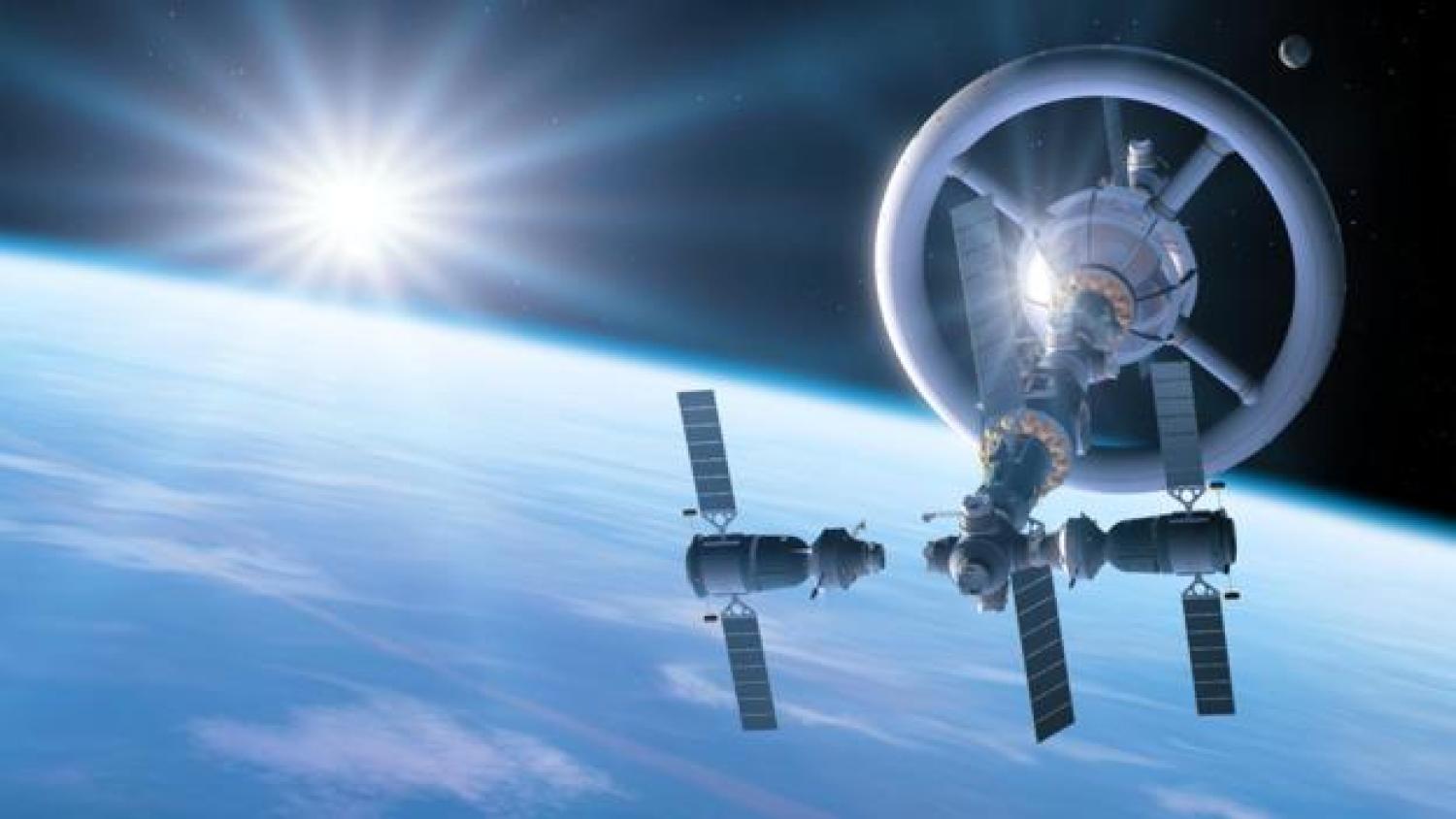
Achievability Contour Display for Lunar Landing
Planetary landing (e.g. on the moon or in the future on Mars) may prove to be the most difficult and critical mission phase. In human missions, whether in a supervisory or manual control mode, the astronauts must identify a suitable landing point that is flat, free of hazards, and near points of scientific interest. Most importantly, they must select a landing point that is achievable given the limited amount of fuel. To assist astronauts with this decision making process we have developed an "achievaiblity limit display" which overlays on a map of the planetary surface the limit which can currently be achieved with the remaining fuel and vehicle state (e.g. altitude, vehicle dyanmics, attitude, surface elevation information, etc.).
We implemented this display in a fixed-base lunar landing simulator at Draper Laboratory and tested in a human-in-the-loop experiment. We found that the achievaiblity display reduced pilot workload, increased situational awareness, and promoted landing closer to points of scientific interest (see Stimpson et al. "Effects of an Achievability Display during Simulated Lunar Landings" 2011). This work was support by NSBRI (PI: Larry Young) and done in collaboration with Alex Stimpson (now at Duke), Kevin Duda at Draper Laboratory, and Larry Young and Charles Oman at MIT. Recent effort have worked on developing a predictive algorithm to precisely identify the limits which can be achieved based upon the vehicle states, guidance laws, and a model of the human operator control inputs.
A Ground-Based Paradigm for Sensorimotor/Neurovestibular Adaptation to Spaceflight
Many phyisological systems (e.g., bone loss, muscle weakening, cardiovascular deconditioning) can be studied here on the ground by using bed rest as an analog environment. Unfortunately, the sensorimotor/neurovestibular changes of spaceflight and return to Earth, do not have a proper ground-based analog. Subjects in bed rest can tilt and move their head, stimulating their vestibular sensory systems in much the same manner as is regularly experienced. We have proposed a "wheelchair head immobilization paradigm" (WHIP) as a method of replicating some of the neurovestibular adaptations to spaceflight. In space, astronauts only experience head translations and rotations, but being in microgravity do not ever experience head tilt relative to gravity like on Earth. This has been hypothesized to drive central reinterpretations of sensory cues, particularly from the otoliths of the vestibular system (Young et al., 1984, Parker et al, 1985, and Merfeld, 2003). We aim to replicate this sensory exposure pattern in a single dimension by securing the subject's head in a custom holder that prevents head tilts relative to gravity. The gravity here on Earth, while impossible to remove, is consistently placed "out of plane". To create pure translation and rotation simulation patterns the subject lays on their side upon and operates a modified, motorized wheelchair device. We hypothesize that within the hours to ~1 day that it typically takes astronauts to undergo neurovestibular adaptation to spaceflight that we will be able to observe similar responses to WHIP exposure. Current developmental efforts are in collaboration with Charles Oman in the Man Vehicle Laboratory (MVL) at MIT.
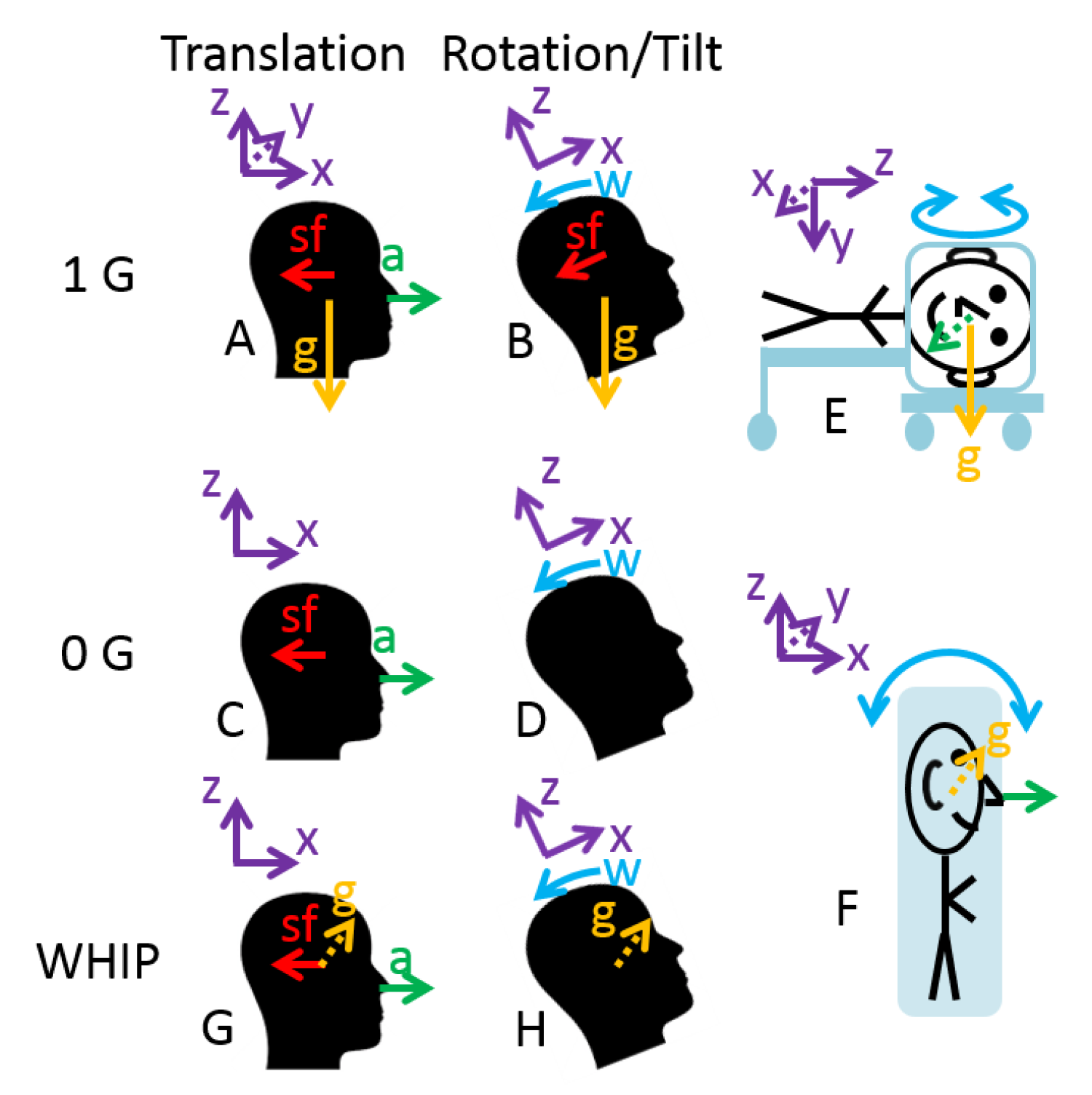
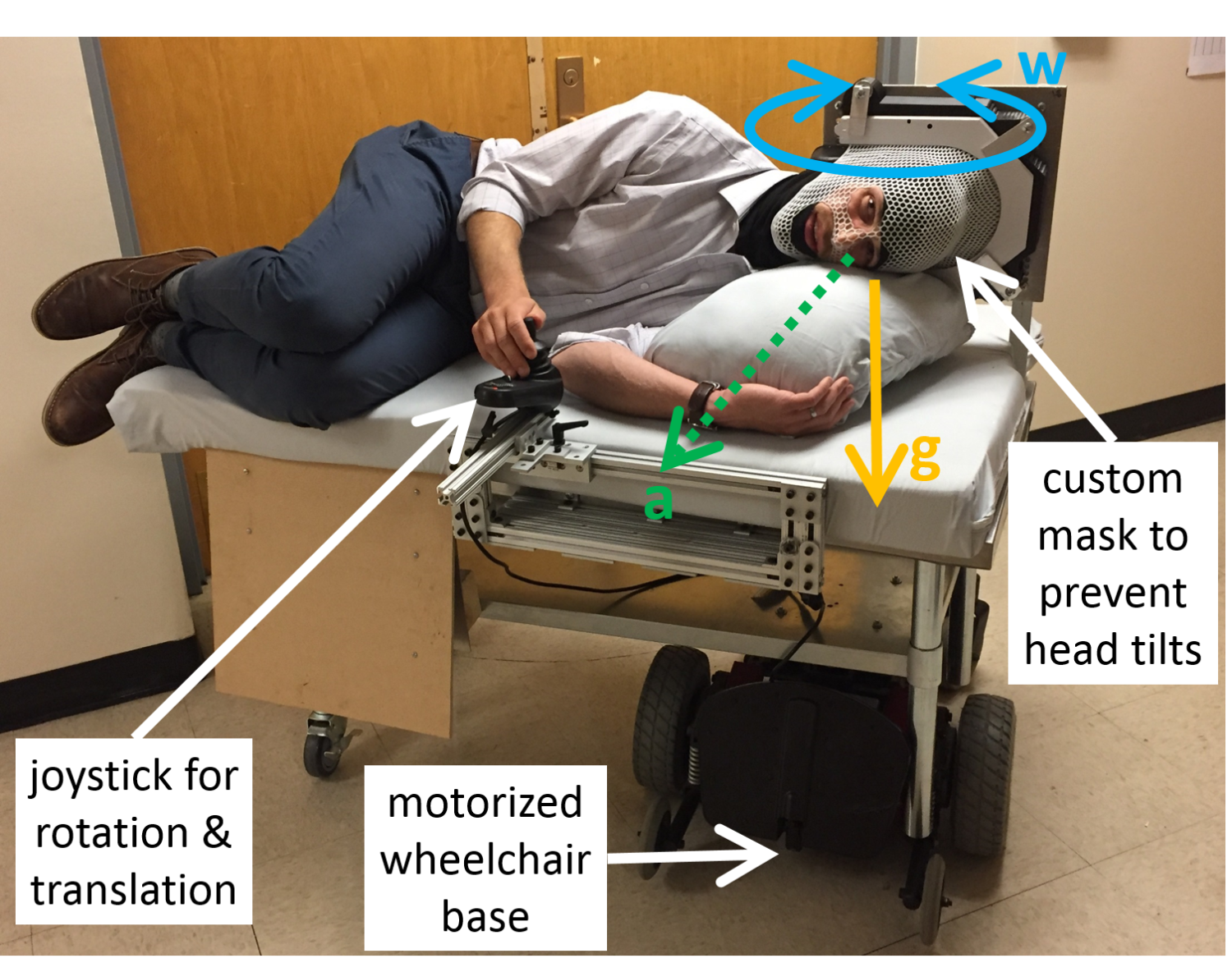
Mathematical Modeling of Human Orientation Perception
Humans sense of orientation perception is one of our most fundamental senses. We perceive our orientation using a combination of multiple sensory cues (e.g., visual, vestibular, somatosensory, proprioceptive, auditory), a process known as "sensory integration". We and others have developed mathematical models of how the brain integrates these multiple sensory cues to yield a central estimate of orientation, rotation, and translation (see Merfeld et al. 1993 "A Multidimensional Model of the Effect of Gravity on the Spatial Orientation of the Monkey" for an early, foundational model). A block diagram of one of our models is shown below.
We apply engineering control system concepts, hypothesizing that that brain uses "internal models" (neural implementations of the dynamics of sensor transduction and physical laws) to provide expected sensory cues, which are then compared to the actual sensory cues (afferent firing rates) to produce "sensory conflict". These sensory conflict signals are weighted to drive our internal sense of orientation state. By inputting actual motions the human experiences (e.g. a lunar landing profile or a complex aircraft flight trajectory), the model can predict the human's perception of orientation. The model is previously well validated for various motion paradigms here in 1 Earth G, but was unable to explain well known perceptual illusions in altered gravity environments. We recently extended the model to predict standard illusions in hyper-G (e.g. greater than 1 Earth G such as would be experienced on a more massive planet or during a coodinated turn in an aircraft). The model can now also make predictions for hypo-G (such as on the on the moon or Mars). (See Clark et al. 2015 "Modeling Human Dynamic Perception of Orientation in Altered Gravity"). In collaboration with Michael Newman at the National Aerospace Training and Research (NASTAR) Center, Charles Oman in the Man Vehicle Laboratory (MVL) at MIT, and Dan Merfeld in the Jenks Vestibular Phsyiology Laboratory at Harvard Medical School/Massachusetts Eye and Ear Infirmary, we are currently supported with a US Army SBIR to further develop and validate this model for aircraft and helicopter applications.
Linear Sled "Hybrid" Approach to Artificial Gravity
As an alternative to the standard centrifuge concept, Jason Gruber (IMSG Laboratories Inc.) has proposed a novel approach for creating artificial gravity. Instead of constantly rotating, the astronaut/rider is linear accelerated on a sled to experience artificial gravity. Since sustained unidirectional acceleration requires exponentially increasingly large displacements, after a brief period of time (e.g., 1 second), the astronaut and chair are quickly rotated 180 degrees, and then decelerated (during which artificial gravitational loading also occurs). The astronaut is then accelerated back towards their original location and the sequence is repeated. The approach creates intermittent artificial gravity during the linear acceleration and deceleration periods that is devoid of the typical problems associated with a centrifuge artificial gravity (vestibular cross-coupling, Coriolis forces, and gravity gradients). In addition, if the 180 degree rotation is done about the astronaut's head, the lower portions of the body will experience centripetal acceleration, hence the "hybrid"-aspect of the paradigm. We are performing a preliminary analysis with a mathematical model of spatial orientation to identify whether the linear sled "hybrid" approach will be disorienting for the riders. Future experiments, in collaboration with Jason Gruber, will investigate whether it is likely to induce motion sickness and then to validate whether the second-to-second intermittent aspect of the approach is effective in reducing or eliminating the physiological deconditioning associated with spaceflight. A video of the paradigm can be found here: https://youtu.be/KTpLVzjvBuc Videos of variants of the concept include: https://youtu.be/nfvczMCvPfs https://youtu.be/HBxjdJObXG0 https://youtu.be/SBh_qO26wQM
Predicting Individual Differences in Sensorimotor Adaptability to Altered Gravity Environments
In space exploration missions, astronauts experience a series of altered gravity environments to which they must adapt their sensorimotor systems. While all astronauts eventually adapt, there appear to be large differences between individuals in how quickly and effectively adaptation occurs, which are poorly understood. Being able to predict these differences is critical to help develop personalized training countermeasures and reduce the likelihood sensorimotor impairment impacts mission success and safety. We have proposed a novel hypothesis that an individual's vestibular perceptual thresholds (i.e. how small of a motion stimulus can be correctly identified as being motion to the left or right), which are a measure of sensory noise, can predict their adaptation capacity. We suggest higher sensory noise levels may slow adaptation because the brain cannot as easily distinguish changes in the environment that require adaptation. We test our hypothesis by trying to correlate an individual's vestibular perceptual thresholds (tested on a 6-dof MOOG platform, left) with their characteristics of adaptation to an altered gravity centrifuge paradigm (tested on a Eccentric Rotator device, right).
Click for some videos of the MOOG and Eccentric Rotator in action! This research is in collaboration with Dan Merfeld in the Jenks Vestibular Phsyiology Laboratory at Harvard Medical School/Massachusetts Eye and Ear Infirmary. The adaptation expeirments are done in collaboration with Faisal Karmali in the JVPL at MEEI and Larry Young in the MVL at MIT. We are also aiming to extend our hypothesis to study astronauts in collaboration with Ajit Mulavara at USRA / NASA Johnson Space Center. This work is supported by the National Space Biomedical Research Institute (NSBRI) as part of the First Award Fellowship program.
Human Perception of Roll Tilt in Hyper-Gravity
To gain a better understanding of how altered gravity (e.g. on the moon or Mars) affects human perception of orientation, we have studied the effects in hyper-gravity. We create a hyper-gravity environment using a long-radius centrifuge. In collaboration with Michael Newman at NASTAR Center, we use the state-of-the-art 25 ft-radius AFTS-400 (PHOENIX) centrifuge (click for a neat video). Once in a particular hyper-gravity, the cab of the centrifuge (with the subject inside) is passively tilted in roll. Subjects report their orientation using a haptic task (aligning a bar with their perceived horizontal) in the dark. We have found that humans overestimate their roll tilt in hyper-gravity, for both static and dynamic roll tilts.
Interestingly, we have found that the amount of overestimation is less for faster tilts, suggesting that when it is reliable, the brain uses information from the semicircular canals to reduce errors in orientation perception. Furthermore, we observed less overestimation on later test sessions suggesting that subjects are adapting to the hyper-gravity environment. (See Clark et al. “Human Perceptual Overestimation of Whole-Body Roll Tilt in Hyper-Gravity” 2015, also here and here for more information online). This work was supported by the NSBRI (PI: Larry Young), the MIT Boeing fellowship, and NASTAR Center.
Human Manual Control in Hyper-Gravity
If humans mispercieve their orientation in altered gravity environments, we hypothesize this will impact their ability to control their orientation. This may be a serious concern, for example, for astronauts piloting a landing vehicle on the moon or Mars. To test this hypothesis, we again used the AFTS-400 (PHOENIX) centrifuge at NASTAR Center. Subjects used a rotational hand controller (i.e. a joystick) which controlled the roll rate of the cab and attempted to null out a psuedo-random disturbance and keep the cab upright (left). Indeed, when first in a hyper-gravity environment subjects performed substantially worse at the nulling task as compared to their performance in normal 1 Earth G (right).
Remarkably, subjects were able to improve their performance in hyper-gravity fairly quickly (time constant of a 100-200 seconds). However these first few hundred seconds may be particularly critical for planetary landing where there is a limited amount of fuel. We further found that subjects who had previously trained in one hyper-gravity level (e.g. 1.5 G) performed substantially better and returned to their 1 G peformance levels more quickly when tested in another hyper-gravity level (e.g. 2 G). This suggests an interesting mechanism called "learning to learn" in which previous adaptation experiences may enhance one's capacity for future adaptation. (See Clark et al. "Human Manual Control Performance in Hyper-Gravity" 2015 and also Clark et al. "Pilot Control and Stabilization of a Rate‑Controlled Vehicle in Hyper-Gravity" 2015). This work was also done in collaboration with Michael Newman at NASTAR Center and supported by supported by the NSBRI (PI: Larry Young), the MIT Boeing fellowship, and NASTAR Center.