Joel Eaves
- Professor
- CHEMISTRY
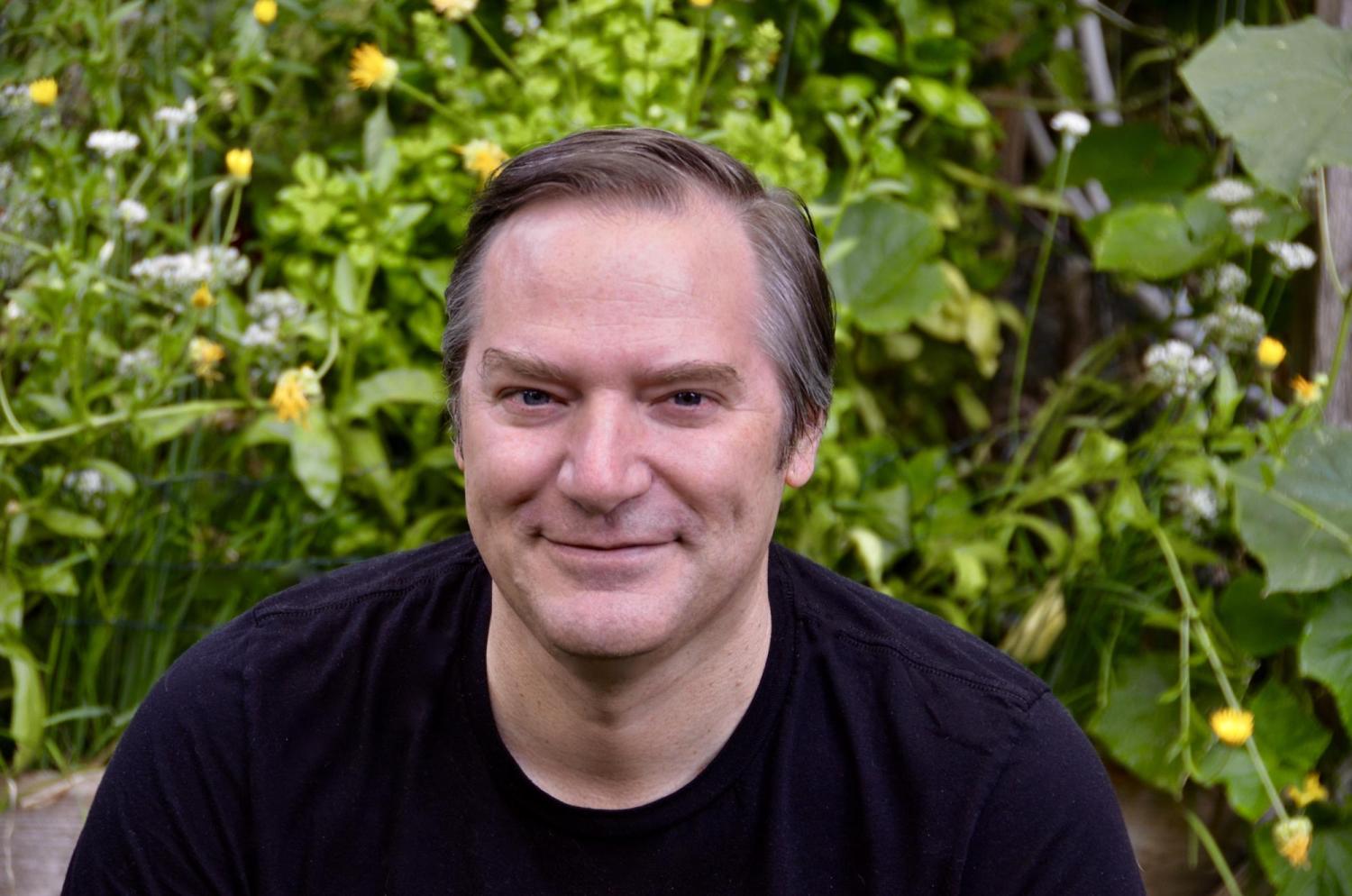
Office: Ekeley W145E
Fax: 303-492-5894
Education
Postdoctoral Scientist: Columbia University (2005-2009)
Ph.D.: Massachusetts Institute of Technology, 2005
B.S. Chemistry, B.S. Physics: University of Wisconsin, Madison (1999)
Areas of Expertise
Nanotechnology/Materials, Physical Chemistry, Theoretical Chemistry, Biophysics, Chemical Physics
The Eaves group works to understand fundamental physical and chemical processes in quantum information, nanoscience, and sustainable chemistry. Our tools are theoretical, where we build mathematical models for complex problems to provide new intuition and physical insights. We often work with experimentalists to interpret existing data, design new measurements, and discover new materials. Research topics include uncovering the molecular structure/function relationships for chemical quantum hardware, creating numerical and theoretical techniques for simulating realistic noise in quantum circuits, converting photons into valuable forms of chemical energy, and modeling water in various chemical and spectroscopic contexts.
- Grimm, R. T. & Eaves, J. D. Direct Numerical solutions to stochastic differential equations with multiplicative noise. arXiv. 2312.05507 (2023).
- Wang, K. et al. Efficient photon upconversion enabled by strong coupling between silicon quantum dots and anthracene. Nat. Chem.15, 1172–1178 (2023).
- Dill, R. D., Smyser, K. E., Rugg, B. K., Damrauer, N. H. & Eaves, J. D. Entangled spin-polarized excitons from singlet fission in a rigid dimer. Nat. Commun.14, 1180 (2023).
- Smyser, K. E. & Eaves, J. D. Singlet fission for quantum information and quantum computing: the parallel JDE model. Sci. Rep.10, 18480 (2020).
- Cline, R. P. & Eaves, J. D. Surface-trapped hole diffusion in CdS and CdSe: the superexchange mechanism. J. Phys. Chem. C.124, 28244–28251 (2020).
- Strong, S. E. & Eaves, J. D. The dynamics of water in porous two-dimensional crystals. J. Phys. Chem. B.121, 189–207 (2016).
- Sweeney, M. C. & Eaves, J. D. Exciton dynamics in carbon nanotubes: from the Luttinger liquid to harmonic oscillators. Phys. Rev. Lett.112, 107402 (2014).