J. Mathias Weber
- Professor
- Institute Fellow, JILA
- CHEMISTRY
- JOINT INSTITUTE FOR LABORATORY ASTROPHYSICS (JILA)
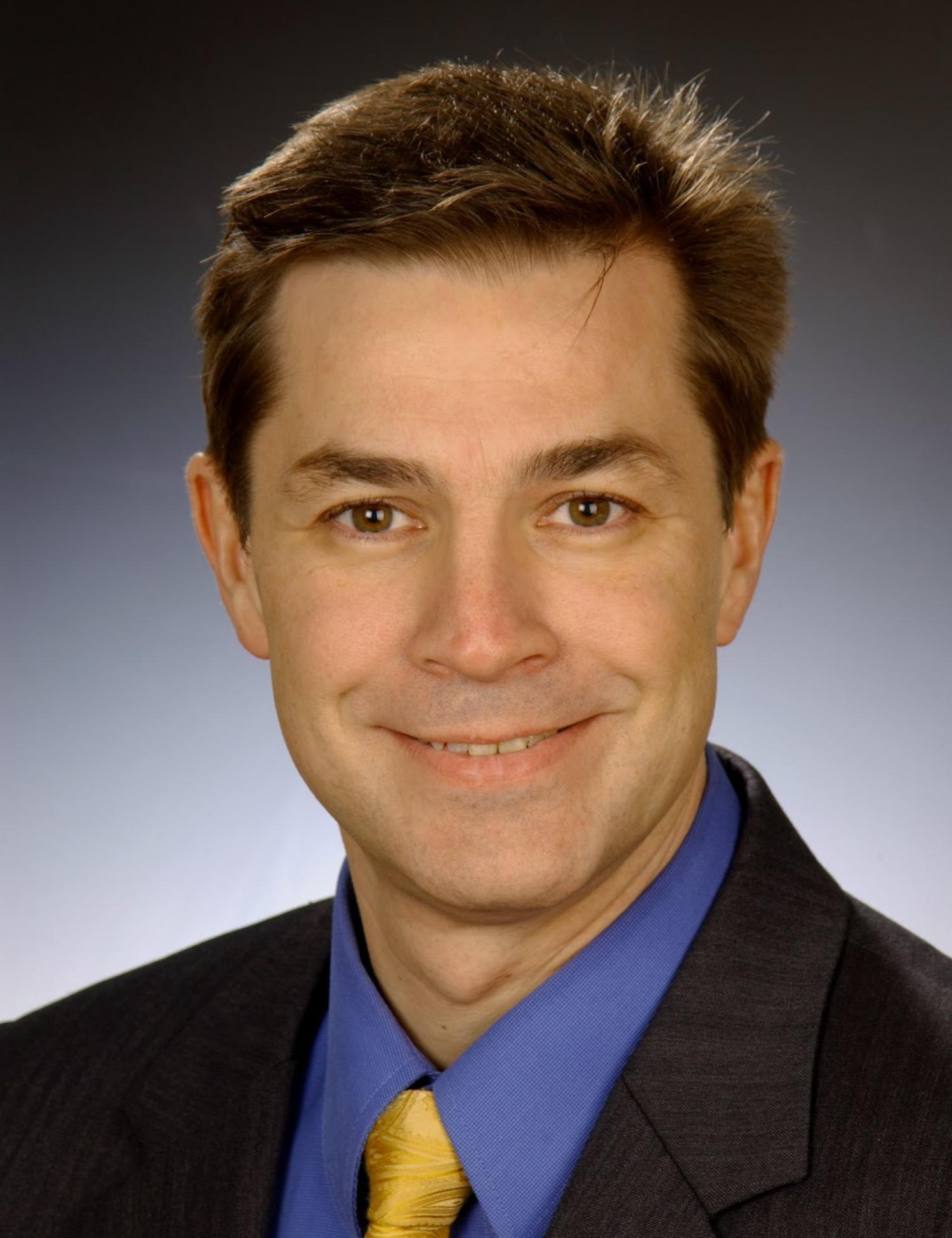
Address
Office: JILA A709
Lab: JILA B113 / B115
Fax: 303-492-5235
Education
Doctoral Degree: University of Kaiserslautern, Germany, 1998
Postdoctoral Associate: Yale University, New Haven, CT (1999-2001)
Habilitation, Venia Legendi for Physical Chemistry: University of Karlsruhe, Germany, 2005
Areas of Expertise
Chemical Physics, Photochemistry, Reaction Dynamics, Nanotechnology/Materials, Renewable Energy
Awards and Honors
Dissertation Award 1999 of the Freundeskreis der Universität Kaiserslautern- Wolfgang-Paul-Award 1999 of the Deutsche Gesellschaft für Massenspektrometrie (German Society for Mass Spectrometry DGMS).- Emmy-Noether-Award of the Deutsche Forschungsgemeinschaft (German Science Foundation), 2002 - 2005- NSF CAREER Award 2009
Please see https://jila.colorado.edu/weberlabs
- L. M. Terry, M. M. Foreman, J. M. Weber, “Effects of Anion Size, Shape, and Solvation in Binding of Nitrate to Octamethyl Calix[4]Pyrrole”, J. Phys. Chem. Lett. 15 (2024) 9481–9486.
- L. M. Terry, M. K. Klumb, D. J. Nemchick, R. Hodyss, F. Maiwald, J. M. Weber, “Cryogenic Ion Vibrational Spectroscopy of Protonated Valine: Messenger Tag Effects”, J. Phys. Chem. A 128 (2024) 7055–7330. https://doi.org/10.1021/acs.jpca.4c03552.
- H. Salzmann, A. B. McCoy, J. M. Weber, “The Infrared Spectrum of Pyrene Anion in the CH Stretching Region”, J. Phys. Chem. A, 21 (2024) 4225–4232. https://pubs.acs.org/doi/10.1021/acs.jpca.4c00966.
- L. M. Terry, M. M. Foreman, A. P. Rasmussen, A. B. McCoy, J. M. Weber, “Probing Ion-Receptor Interactions in Halide Complexes of Octamethyl Calix[4]Pyrrole”, J. Am. Chem. Soc. 146 (2024) 12401–12409. https://doi.org/10.1021/jacs.3c13445.
- H. Salzmann, A. P. Rasmussen, J. D. Eaves, J. M Weber, “Competition Between Water-Water Hydrogen bonds and Water-π Bonds in Pyrene-Water Cluster Anions”, J. Phys. Chem A 128 (2024) 2772 – 2781. https://doi.org/10.1021/acs.jpca.4c00997.
- M. M. Foreman, J. M. Weber, “Ion Binding Site Structure and the Role of Water in Alkaline Earth EDTA Complexes”, J. Phys. Chem. Lett. 13 (2022) 8558–8563. https://pubs.acs.org/doi/full/10.1021/acs.jpclett.2c02391.
- J. C. Beimborn II, W. Zagorec-Marks, J. M. Weber, "Spectroscopy of Resonant Intermediate States for Triplet-Triplet Annihilation Upconversion in Crystalline Rubrene – Radical Ions as Sensitizers", J. Phys. Chem. Lett. 11 (2020) 7212-7217. https://doi.org/10.1021/acs.jpclett.0c01999.
- J. C. Beimborn II, L. R. Walther, K. D. Wilson, J. M. Weber, "Size-Dependent Pressure-Response of the Photoluminescence of CsPbBr3 Nanocrystals", J. Phys. Chem. Lett. 11 (2020) 1975-1980. https://doi.org/10.1021/acs.jpclett.0c00174.
- W. Zagorec-Marks, M. M. Foreman, J. R. R. Verlet, J. M. Weber, "Probing the Microsolvation Environment of the Green Fluorescent Protein Chomophore in Vacuo", J. Phys. Chem. Lett. 11 (2020) 1940-1946. https://doi.org/10.1021/acs.jpclett.0c00105.
- L. G. Dodson, M. C. Thompson, J. M. Weber "Characterization of Intermediate Oxidation States in CO2 Activation" Annu. Rev. Phys. Chem. 69 (2018) 231–252. https://doi.org/10.1146/annurev-physchem-050317-021122.